Solar Energy, Latitude and Coastal Plant Diversity for Management of Sand Dune Parks
Department of Biology, Kunsan National University, Korea
Department of Envionmental Education, Mokpo National University, Korea
Department of Biological Science, Mokpo National University, Korea keco@mokpo.ac.kr
Institution for Marine and Island Cultures, Mokpo National University, Korea
Korea Environmental Technology Consulting Hotline, Korea
Division of Ecosystem Research, National Park Research Institute, Korea
Division of Ecological Assessment, National Institute of Ecology, Korea
Department of Biological Science, Mokpo National University, Korea
Abstract
This study attempted to find consistent relationships between climate, latitude, and the richness of plant species on sand dunes. The richness of plant species on sand dunes in the Northern and the Southern hemisphere decreased with latitude or increased with air temperature. This suggests a latitudinal gradient of vascular plant richness on sand dunes in both hemispheres. However, the relationships between the richness of coastal plant species and mean annual precipitation were not statistically significant in either hemisphere. There is a vital need for construction of sand dune parks having conservation programs.
Keywords
Global climate, sand dunes, latitude, solar energy, plant diversity
Introduction
Coastal dunes are eolian landforms that develop in coastal situations where an ample supply of loose, sand-sized sediment is available to be transported inland by the ambient winds (Martinez et al. 2008, Doody 2013). They are unique ecosystems at the spatial transition between terrestrial and marine environments. Coastal dunes are part of the sand-sharing system composed of the highly mobile beach and the more stable dune. A large variety of coastal dune forms are found inland from and above the storm-water level of sandy beaches, and occur on ocean, and estuary shorelines. However, many factors that affect coastal dunes, including geomorphology, sand texture, water extraction, trampling, invasive species, grass encroachment, sea-level rise, and climate change, are happening worldwide (Barbour et al. 1987, Castillo et al. 1991, 1996, Musila et al. 2001, Forcy et al. 2008, Houle 2008, Yu et al. 2008, Fenu et al. 2012, Moeslund et al. 2013).
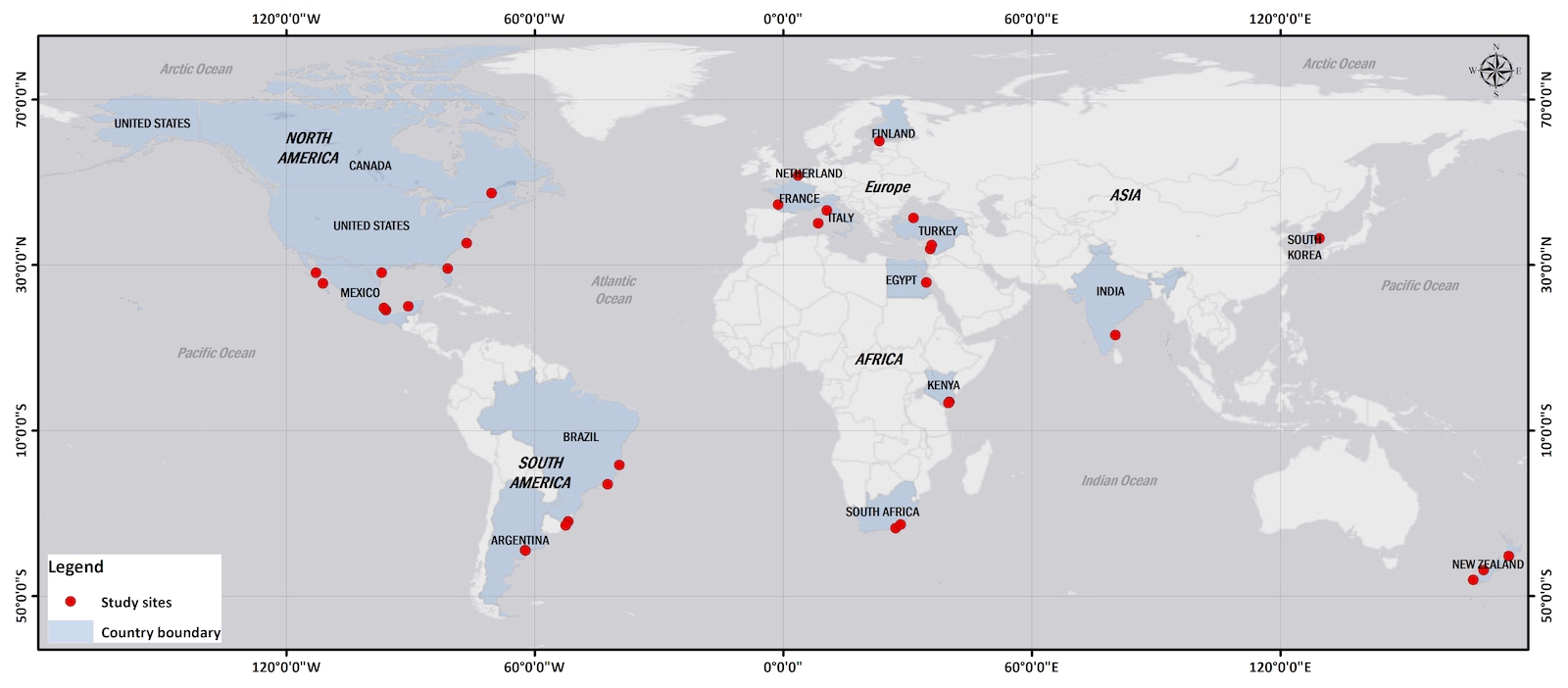
Environmental factors and species diversity
Many plant and animal species dwell in the tropics and richness of species increases with decreasing latitudes (Currie 1991, Gaston 2000, Ohlemüller and Wilson 2000, Huston et al. 2003). An equatorward increase in the richness of species is often paralleled by a decrease in the mean latitudinal range of species. A peak in the richness of species is predicted at tropical latitudes (Colwell & Hurtt 1994, Cordazzo and Seeliger 1988, Costa et al. 1996). Recent studies have addressed latitudinal gradients in species diversity and their explanations, including Rapoport's rule (Currie 1991, Rhode 1999, Gaston 2000, Hawkins et al. 2003, Wang et al. 2009): Stevens (1989) proposed Rapoport’s rule as a possible explanation for the higher richness of species of animals and plants in the tropics. The rule postulates that tropical species have smaller ranges on average than do temperate taxa because of the tolerance of temperate species to a broader range of environmental conditions. Rapoport’s rule has been applied to some groups in some continents. But the exceptions to the rule are numerous and its general applicability has been seriously questioned (Francis et al. 2003, Gaston 2000).
Some models relate plant richness to mean annual temperature, annual water deficit, and their interaction. Other models relate richness to annual potential evapotranspiration (PET) and water deficit. Both are globally consistent, very strong, and independent of the diverse evolutionary histories and functional assemblages of plants in different parts of the world (Francis and Currie 2003). Patterns of the richness of plant species in terms of resource availability and of direct physiological effects of resource availability on plant growth have been described (Pausas and Austin 2001). They suggest that there are a variety of patterns of the richness of species along environmental gradients.
An increase in the richness of plant and animal species correlates with net primary productivity (NPP) by means of several potential mechanisms, such as complementarity of resource use and positive interspecific interactions, which implies that an increase in the availability of resources favors increased diversity (Morin 2000, Ihm et al. 2012). At the global scale of investigation, NPP is a measure of resource availability for plants and is determined by geographic variations in climatic and edaphic characteristics, and can be readily simulated.
Hawkins et al. (2003) tried to document the support for the climatically based energy hypothesis, and evaluated two versions of the hypothesis, that is, the productivity and ambient energy hypotheses. Focusing on studies extending over 800 km, they found that measures of energy, water, or water-energy balance could explain spatial variation in richness better than could other climatic and non-climatic variables (Gaston 2000, Hawkins et al. 2003, Whittaker et al. 2007). Even when considered individually and in isolation, water-energy variables explain on average over 60% of the variation in the richness of a wide range of plant and animal groups. Furthermore, water variables usually represent the strongest predictors in the tropics, subtropics, and warm temperate zones, whereas energy variables (for animals) or water-energy variables (for plants) dominate in high latitudes. The results indicate that the direct or indirect interaction between water and energy via plant productivity largely explains the globally extensive plant and animal diversity gradients, but that for animals there also is a latitudinal shift in the relative importance of ambient energy vs. water moving from the poles to the equator.
Recent large-scale studies have demonstrated that geographic gradients in the richness of plants, in particular of woody plants, such as trees and shrubs, can be viewed as by-products of water-energy dynamics (O'Brien et al. 2000, Wang et al. 2009). A two-variable model capturing the dynamic relationship between energy (heat: light) and water (rainfall) accounts for most of the variation in woody plant richness across southern Africa at species, genus, and family levels.
Coastal dunes and species diversity
Widespread distribution of coastal dunes is coincident with the worldwide occurrence of wave-dominated sandy beaches and with coastal barrier systems. Further, coastal dunes exist more extensively where areas of persistent coastal sand supply and dominant onshore winds favor episodes of inland transport to create broad dune fields. Active as well as stabilized coastal dunes of various scales and morphologies are recognized as products of coastal dynamics (Huston et al. 2003, Martínez et al. 2008). Flora data and environmental factor data with relatively even distributions from coastal sand-the dune areas of northern and southern hemispheres were studied (Gaston 2000, Hesp 2004, Myeong 2010, Lee et al. 2018, Lee and Kim 2018). The richness of plant species on sand dunes in the Northern and Southern hemispheres decreased with latitude and increased with solar energy (air temperature), which had high multicollinearity and suggests a latitudinal gradient of vascular plants in both hemispheres (Esler 1970, Craig 1975, van der Valk 1975, Johnson 1977, van der Laan 1979, Hay et al. 1981, Johnson 1982, Lubke 1983, Barbour et al. 1987, Sykes and Wilson 1987, Cordazzo and Seeliger 1988, Moreno-Casasola 1988, Sykes and Wilson 1991, Avis 1995, Castillo and Moreno-Casasola 1996, Costa et al. 1996, Hellemaa 1998, Jung and Kim 1998, Musila et al. 2001, Fontana 2005, Forey et al. 2008, Houle 2008, Yu et al. 2008, Ciccarelli et al 2012, Fenu et al. 2012, Monserrat et al. 2012, Giaretta et al. 2013, Fig. 2). However, the relationships between the richness of coastal plant species and mean annual precipitation were not significant in either hemispheres (Fig. 2).
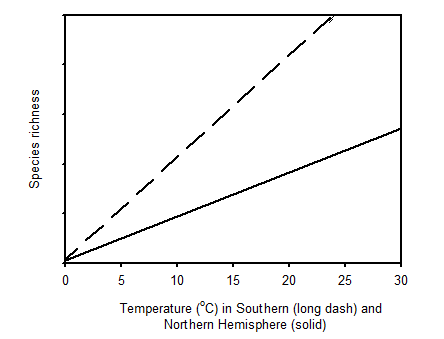
Discussion
To explain latitudinal gradients in species diversity, several hypotheses have been reviewed (Stevens 1989, Rhode 1992, Gaston 2000, Ohlemüller and Wilson 2000, Huston et al. 2003). Some hypotheses are based on the assumption that some taxa have greater diversity in the tropics. Explanations include different degrees of interactions, epiphyte load, biotic spatial heterogeneity, host diversity, niche width, population growth rate, environmental harshness, and patchiness at different latitudes. However, other explanations are not supported by sufficient evidence (Rhode 1992). For example, correlations between species diversity, environmental stability and predictability, productivity, abiotic rarefaction, physical heterogeneity, seasonality, and number of habitats are inconsistent. Ecological and evolutionary time hypotheses that communities will diversify in time cannot explain species diversity gradients. Only difference in solar energy was consistently correlated with diversity gradients along latitude and elevation (Fig. 2).
Although many factors are likely to be responsible for local differences in diversity and secondary effects on latitudinal gradients, the primary cause appears to be the effect of temperature (solar energy) on evolutionary speed (Rhode 1992, Morin 2000, Allen et al. 2002, Evans et al. 2005, Ihm et al. 2012). The widespread view that diversity is limited by solar energy is based on an unsupported belief that all habitats are filled to their full capacity. However, saturation of habitats does not rule out a further increase in the number of species by subdivision of niches. Accumulating evidence supports the view that many vacant niches exist, particularly for parasites and symbionts. Evolution is gradually filling these niches because speciation happens faster than extinction and there is greater "effective" evolutionary time in the tropics, because greater evolutionary speed has led to greater numbers of species in the tropics. It is likely that habitats at all latitudes could support more species than presently exist (and perhaps equal numbers at all latitudes). Paradoxically, the tropics have more extant vacant niches than do colder regions, because a greater number of free-living species provide more opportunities for "dependent" species such as parasites. Efforts to find equilibrium explanations for these gradients have led to numerous ecological studies on factors influencing species diversity.
Areas with energy have also often been shown to correlate strongly with the richness of species at a regional scale. The area effect is most evident in studies of archipelagoes and other habitats where area varies widely, while other environmental conditions are reasonably homogeneous (Connor and McCoy 1979, Lee et al. 2018). Area and energy both undoubtedly affect regional richness. The strength of correlation between richness and area or energy depends strongly on their range of variability. In Wright's (1983) species-energy model, richness on islands is hypothesized to depend on area times areal energy flux (i.e., actual evapotranspiration (AET), PET, or primary production). Energy flux per unit of area and area itself are therefore postulated to affect richness proportionally. A doubling of either area or energy should lead to the same increase in richness.
It has long been known that the diversity of most taxonomic and functional groups is the highest in the tropics. This has usually been attributed to higher productivity (resource availability) or reduced seasonality rather than to the kinetic effect of higher temperatures (Rohde 1992, Gaston 2000, Hawkins et al. 2003, Brown et al. 2004, Whittaker et al. 2007). Allen et al. (2002) and Brown et al. (2004) have shown that the richness of species in many groups of plants and animals has the same Boltzmann relationship with environmental temperature that metabolic rate does. This result holds true not only along latitudinal gradients, but also along elevation gradients, where variables such as photon flux, seasonal changes in day length, and biogeographic history are held relatively constant. The implication is that much of the variation in species diversity is directly attributable to the kinetics of biochemical reactions and ecological interactions. The highest diversity on earth is found in warm and productive environments, such as tropical rain forests, coral reefs, and sand dunes, where the kinetics of interactions might lead to rapid exclusion (Gaston 2000, Allen et al. 2002, Hawkins et al. 2003). In the recently proposed metabolic theory of ecology, the metabolic rate of organisms governs ecological patterns and processes, including geographical patterns of the richness of species in terms of the effect of temperature and body size on metabolism of organisms (O'Brien et al. 2000, Wang et al. 2009).
Is it possible to preserve the form and function of coastal dunes such as species diversity? (Costa et al. 1996, Heslenfeld et al. 2004, Martínez et al. 2008). Based on current global trends, the future looks bleak. For example, the following have been projected: (1) agriculture will intensify in these sandy and well-drained habitats; (2) pressure from tourism will increase; and (3) sea level rise will displace the beach-dune system and narrow the protected dune zone, while erosion rates will be exacerbated. Thus, it seems that human development along the coast will continue. It would be wise to do so in a proper and sustainable manner. This necessarily implies agreement with the philosophical supposition that conservation and development are not irreconcilable. Efforts must be made to strike a balance between them. Because coastal defense mechanisms that fail to consider natural processes are highly costly and ineffective, management towards maintaining the dynamics of coastal-dune species diversity should be promoted. In fact, it is known that the more diverse the system, the better it is able to withstand change. The global sand-dune system with diverse species and vegetation is more resilient to changing conditions. Thus, application of the results of this research, coupled with proper management and conservation policies, will sustain these environments and maintain higher species diversity.
Conclusion
We studied the relationships between environmental factors such as latitude, solar energy and the richness of coastal plant species on global coastal sand dunes. Solar energy and latitude was evaluated in a globally consistent manner. The relative importance of solar energy or latitude has determined the richness of plant species of coastal habitats, and it needs further study in various ecosystems. There is a vital need for management and construction of sand dune parks having conservation programs, such as Long Term Ecological Research (LTER) program.
Acknowledgements
This research was supported by the Basic Science Research Program through the National Research Foundation of Korea (NRF) funded by the Ministry of Education (NRF-2018R 1D 1A 1B06043748).
References
- Allen AP, Brown JH, Gillooly JF. (2002) Global biodiversity, biochemical kinetics and the energy equivalence rule. Science 297: 1545–1548.
- Avis AM. (1995) An evaluation of the vegetation developed after artificially stabilizing South African coastal dunes with indigenous species. Journal of Coastal Conservation 1: 41-50.
- Barbour G, Rejmánek M, Johnson F, Pavlik BM. (1987) Beach vegetation and plant distribution patterns along the northern Gulf of Mexico. Phytocoenologia 15: 201–233.
- Brown JH, Gillooly JF, Allen AP, Savage VM, West GB. (2004) Toward a metabolic theory of ecology. Ecology 85: 1771–1789.
- Castillo SA, Moreno-Casasola P. (1996) Coastal sand dune vegetation: an extreme case of species invasion. Journal of Coastal Conservation 2: 13-22.
- Chae YA, Ku JO, Seo HS, Lee YM, Jeong SK. (2012) Basic biostatistics. Hyangmunsa Press, Seoul.
- Ciccarelli D, Bacaro G, Chiarucci A. (2012) Coastline dune vegetation dynamics, Evidence of no stability. Folia Geobot 47: 263–275.
- Colwell RK, Hurtt GC. (1994) Nonbiological gradients in species richness and a spurious Rapoport effect. American Naturalist 144: 570-595.
- Connor EF, McCoy ED. (1979) The statistics and biology of the species-area relationship. American Naturalist 113: 791-833.
- Cordazzo CV, Seeliger U. (1988) Phenological and biogeographical aspects of coastal dune plant communities in southern Brazil. Vegetatio 75: 169-173.
- Costa CSB, Cordazzo CV, Seeliger U. (1996) Shore disturbance and dune plant distribution. Journal of Coastal Research 12: 133-140.
- Craig RM. (1975) Natural vegetation on Florida's coastal dunes. Proceedings of the Soil and Crop Society of Florida 34:169–171.
- Currie DJ. (1991) Energy and large scale patterns of animal and plant species richness. American Naturalist 137: 27-49.
- Doody JP. (2013) Sand dune conservation, management and restoration. Springer, New York.
- Esler AE. (1970) Manawatu sand dune vegetation. Proceedings of the New Zealand Ecological Society 17: 41-46.
- Evans KL, Greenwood JJD, Gaston KJ. (2005) Dissecting the species-energy relationships. Proc R Soc B, 272: 2155–2163.
- Fenu G, Cogoni D, Ferrara C, Pinna MS, Bacchetta G. (2012) Relationships between coastal sand dune properties and plant community distribution: the case of Is Arenas (Sardinia). Plant Biosystems 146: 586-602.
- Fontana SL. (2005) Coastal dune vegetation and pollen representation in south Buenos Aires Province, Argentina. Journal of Biogeography 32: 719–735.
- Forey E, Chapelet B, Vitasse Y, Tilquin M, Touzard B, Michalet R. (2008) The relative importance of disturbance and environmental stress at local and regional scales in French coastal sand dunes. Journal of Vegetation Science 19: 493-502.
- Francis AP, Currie DJ. (2003) A globally-consistent richness-climate relationship for angiosperms. American Naturalist 161: 523-536.
- Gaston KJ. (2000) Global patterns in biodiversity. Nature 405: 220-227.
- Giaretta A, Menezes LFT, Pereira OJ. (2013) Structure and floristic pattern of a coastal dunes in southeastern Brazil. Acta Botanica Brasilica 27: 87-107.
- Hawkins BA, Field R, Cornell HV, Currie DJ, Guegan JF, Kaufman DM, Kerr JT, Mittelbach GG, Oberdorff T, O'Brien EM, Porter EE, Turner JRG. (2003) Energy, water, and broad-scale geographic patterns of species richness. Ecology 84: 3105-3117.
- Hay JD, Henriques RPB, Lima DM. (1981) Quantitative comparisons of dune and foredune vegetation in resting ecosystems in the State of Rio de Janeiro, Brazil. Rev. Brasil. BioI. 41: 655-662.
- Hellemaa P. (1998) The development of coastal dunes and their vegetation in Finland. Fennia 176: 111-221.
- Heslenfeld P, Jungerius PD, Klijin JA. (2004) European coastal dunes: ecological values, threats, opportunities and policy development. In Coastal dune, ed. Martínez M.L., and N.P. Psuty N.P. pp. 335-351. Springer-Verlag, New York.
- Hesp PA. (2004) Coastal dunes in the Tropics and Temperate regions: location, formation, morphology and vegetation processes. In Coastal dune, ed. Martínez M.L., and N.P. Psuty N.P. pp. 29-49. Springer-Verlag, New York.
- Houle G. (2008). Plant species richness and its determinants on a coastal dune system at Iles de la Madeleine, Québec (Canada). Ecoscience 15: 113-120.
- Huston MA, Brown JH, Allen AP, Gillooly JF. (2003) Heat and biodiversity. Science 299: 512-513.
- Ihm B-S, Lee J-S, Kim J-W. (2012) Global warming and biodiversity model projections. J Ecol Env (J Field Biol) 35: 157-166.
- Johnson AF. (1977) A survey of the strand and dune vegetation along the Pacific and southem gulf coasts of Baja California, Mexico. Journal of Biogeography 7: 83-99.
- Johnson AF. (1982) Dune vegetation along the eastern shore of the Gulf of California. Journal of Biogeography 9: 317-330.
- Jung Y-K, Kim J.W. (1998) Coastal sand dune vegetation in Kyungpook province. J Ecol Env(Korean J Ecol) 21: 257-262.
- Lee J-S, Choi S-W, Kim J-W, Lee SH, Son DH, Park M-W. (2018) Coastal plant species richness in sand dunes along temperature in Northern and Southern hemispheres. Proceeding of 2018 Korean Ecology-Environment Science Conference. pp. 154
- Lee J-S, Kim J-W. (2018) Dynamics of zonal halophyte communities in salt marshes in the world. Journal of Marine and Island Cultures 7(1): 84-106.
- Lubke RA. (1983) A survey of the coastal vegetation near Port Alfred, Eastern Cape. Bothalia 14: 725-738.
- Martínez ML, Psuty NP, Lubke RA. (2008a) A perspective on coastal dunes. In Coastal dunes, ed. Martínez M.L., Psuty N.P. pp. 3-10. Springer-Verlag, New York.
- Martínez ML, Maun MA, Psuty NP. (2008b) The fragility and conservation of the world’s coastal dunes: Geomorphological, ecological and socioeconomic perspectives. In Coastal dunes, ed. Martínez M.L., Psuty N.P. pp. 355-369. Springer-Verlag, New York.
- Moeslund JE, Arge L, Bocher PK, Dalgaard T, Svenning JC. (2013) Topography as a driver of local terrestrial vascular plant diversity patterns. Nordic Journal of Botany 31: 129–144.
- Monserrat AL, Celsi CE, Fontana SL. (2012) Coastal dune vegetatìon of the Southern Pampas (Buenos Aires, Argentìna) and its value for conservatìon. Journal of Coastal Research 28: 23-35.
- Moreno-Casasola P. (1988) Patterns of plant species distribution on coastal dunes along the Gulf of Mexico. J Biogeog 15: 787–806.
- Morin PJ. (2000) Biodiversity's ups and downs. Nature 406: 463-464.
- Musila WM, Kinyamario JI, Jungerius PD. (2001) Vegetation dynamics of coastal sand dunes near Malindi, Kenya. Afr J Ecol 39: 170-177.
- Myeong HH. (2010) On conservation and management plan of coastal sand dune ecosystem using health assessment. Mokpo National University, Ph.D. dissertation.
- O'Brien EM, Field R, Whittaker RJ. (2000) Climatic gradients in woody plant (tree and shrub) diversity: water-energy dynamics, residual variation, and topography. Oikos 89: 588-600.
- Ohlemüller R, Wilson JB. (2000) Vascular plant species richness along latitudinal and altitudinal gradients: a contribution from New Zealand temperate rainforests. Ecology Letters 3: 262-266.
- Pausas JG, Austin MP. (2001) Patterns of plant species richness in relation to different environments: An appraisal. Journal of Vegetation Science 12: 153-166.
- Rohde K. (1992) Latitudinal gradients in species diversity, the search for the primary cause. Oikos 65: 514-527.
- Rohde K. (1999) Latitudinal gradients in species diversity and Rapport's rule revisited: a review of recent work and what can parasites teach us about the causes of the gradients? - Ecography 22: 593-613.
- Stevens GC. (1989) The latitudinal gradient in geographical range: How so many species coexist in the tropics. American Naturalist 133: 240-256.
- Sykes MT, Wilson JB. (1987) The vegetation of a New Zealand dune slack. Vegetatio 71: 13-19.
- Sykes MT, Wilson JB. (1991) Vegetation of a coastal sand dune system in southern New Zealand. Journal of Vegetation Science 2: 531-538.
- van der Laan D. (1979) Spatial and temporal variation in the vegetation of dune slacks in relation to the ground water régime. Vegetatio 39: 43-51.
- van der Valk AG. (1975) The floristic composition and structure of foredune plant communities of Cape Hatteras National Seashore. Chesapeake Science 16: 115-126.
- Wang Z, Brown JH, Tang Z, Fang J. (2009) Temperature dependence, spatial scale, and tree species diversity in eastern Asia and North America. PNAS 106: 13388-13392.
- Whittaker RJ, Nogués-Bravo D, Araújo MB. (2007) Geographical gradients of species richness: a test of the water-energy conjecture of Hawkins et al. (2003) using European data for five taxa. Global Ecol Biogeogr 16: 76–89.
- Yu S, Bell D, Sternberg M, Kutiel P. (2008) The effect of microhabitats on vegetation and its relationships with seedlings and soil seed bank in a Mediterranean coastal sand dune community. Journal of Arid Environments 72: 2040–2053.