Ecology of ancient Tank Cascade Systems in island Sri Lanka
Review
Department of Plant Sciences, Faculty of Agriculture, Rajarata University of Sri Lanka, Puliyankulama, Anuradhapura 50000, Sri Lanka nalakagee@gmail.com
Department of Crop Science, Faculty of Agriculture, University of Peradeniya, Peradeniya 20400, Sri Lanka pkumaralk@yahoo.com
Abstract
Sri Lanka has vast dry low-lying plains irrigated using traditional micro-(or meso-) watershed management system referred to as the Tank Cascade System (TCS). We discuss the implications of this irrigation system which include the development of lowland paddy (Oryza sativa L.) farming. In addition, the TCS has retarded negative consequences from chronic and recurrent droughts, seasonal flooding, land degradation and enhanced the food security while helping to attain self sufficiency in rice. The indirect benefits from TCS include numerous environmental services from these naturalized habitats.
Keywords
Tank Cascade System, Watershed management
Introduction
Sri Lanka is an oceanic tropical island in the Indian sub-continent and located at 5°54′–9°52′N 79°39′–81°53′E (Fig. 1). The total land extent of the country is 6,570,134 ha which consist of central highlands with an altitude more than 2500 m above mean sea level. The central mountain ranges are surrounded by broad lowland plains which include both dry and wet climates. The population of the country is 21 million at present with a population density of 333 persons/km2 and the percent growth of population has been declined to 1–1.3 during the last decade (CBSL, 2012).
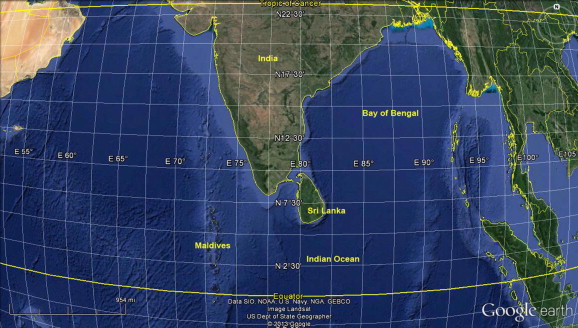
The manipulation of water resources is a fundamental aspect of the long term agricultural sustainability in any civilization. Water collection techniques differ across countries according to the nature of the topography, importance of wetness or aridity, the nature of soils and the usage of collected water. For example in Sri Lanka, water is collected using man-made tanks in dry areas mainly for paddy cultivation (Brohier, 1935). These tanks comprise of various components of ecological importance (detailed in section “Zones and components of a TCS”). In Middle East and Pakistan, desert sand tanks are used in arid areas (UNCCD, 2005). A desert sand tank consists of an embankment built across a stream bed which is firmly bound to the bed rock and canal walls. The UNCCD (2005) detail various technologies for water harvesting and conservation, detention, dispersion and diversion structures and/or their modifications from dry land areas.
The concept of the tank cascade was first coined by Madduma Bandara (1995) to explain the network of small tanks to large reservoirs that still exist in modern-day Sri Lanka. These ancient irrigation structures were evolved with the advancement of traditional knowledge over two millennia and still act as essential elements of water management for agriculture in the dry zone of Sri Lanka. The main principle behind the Tank Cascade Systems (TCSs) is recycling and re-use of water through a network of small to large scale tanks. Brohier (1935) has also reported village tanks and their association with ancient irrigation works in Sri Lanka. The ‘tank’ is the direct translation of the Sinhala word ‘wewa’. In contrast to “an artificial pool, lake or reservoir”, there are fundamental differences between a wewa and a man-made tank such as the tree interceptor and waterhole in the inlet (detailed in section “Zones and components of a TCS”). Henceforth, the word tank refers to the traditional wewa in Sinhala. A ‘cascade’ is a connected series of tanks organized within a micro-(or meso-) catchment of the dry zone landscape for storing, conveying and utilizing water from an ephemeral rivulet (Madduma Bandara, 1995) (Fig. 2). Each of these cascades delineates a distinct small watershed or meso-catchment ranging in extent from 13 to 26 km2 with an average of 20 km2.
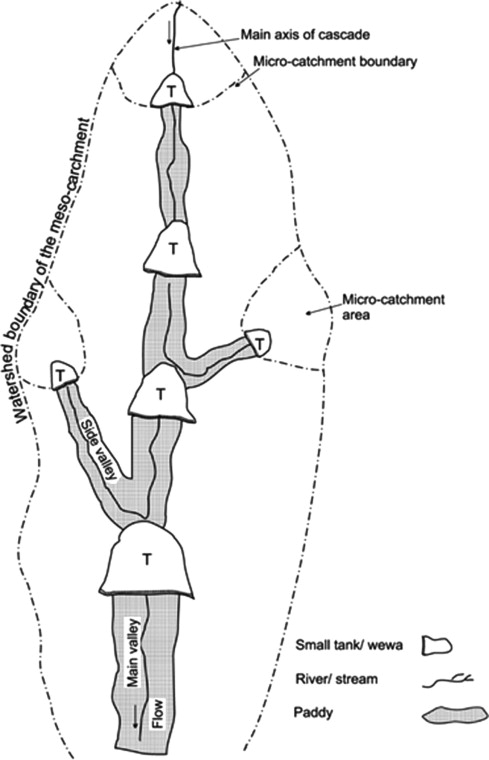
An island is a discrete land unit smaller than a continent which is surrounded by water (or by an unsuitable habitat in Biogeography) and a group of islands is called an archipelago. Some islands, which rise up from the ocean floors, are oceanic, whereas some of them, which submerge from a continental shelf, are continental. Initial civilization of Sri Lanka dates back to 543 BC with the arrival of Prince of Vijaya from North India. He initially married to Kuveni, a woman from an indigenous tribe, and Vijaya became the first King of Sri Lanka. He colonized in riverbanks in the dry zone of Sri Lanka. The earliest medium-scale irrigation reservoir (surface area of 107 ha) that can be identified with certainty, which was built in 300 BC, and remains operational, is the Basavakkulama tank in the World Heritage city of Anuradhapura. Thereafter, about 30,000 tanks have been built in a land area of about 40,000 km2 in the dry zone (Mendis, 2003; Marambe et al., 2012). Some of them have been in operation continuously for more than 2000 years (Brohier, 1935). Approximately 7620 small tanks with an irrigated area of 0–80 ha are operational at present (Panabokke et al., 2002). This indicates that the TCSs of Sri Lanka evolved over two millennia. Therefore, they are now naturalized and bound to the dry zone landscape in Sri Lanka. As well as providing irrigation water, TCSs have impacted the microclimate by creating cooler habitats, enhanced the biodiversity and agrobiodiversity, and paved the way for a unique decentralized social system in Sri Lanka where farmers have held the highest social rank (Marambe et al., 2012). It is suggested that 3rd to 7th century and 12th century have been the period where ancient irrigation technology was peaked in the country (Parker, 1907). This paper reviews lessons from this time tested case of TCSs and provide the way forward for dry land management in the 21st century. First we outline underline reasons for developing TCSs and second ecological implications, components and different zones in TCSs are presented. Management systems and the cultural diversity are described in the latter and finally we identify historic and modern challenges to provide insights water management under modern climate change scenarios.
Why develop Tank Cascade Systems?
The dry zone covers much of lowlands of Sri Lanka and receives an annual rainfall lesser than 1750 mm (Punyawardane, 2008) (Fig. 3). Annual evaporation ranges between 1700 and 1900 mm which results in a soil moisture deficit during dry periods (Panabokke et al., 2002). This island in the Indian Ocean, has a tropical monsoonal climate which is characterized by a long dry spell (nearly 8 months in some cases from Feb. to Oct.) and short rainy season (nearly 4 months from Sep. to Jan.), during which 80% of the annual rainfall is received. The dry season in the dry zone is plagued by chronic and recurrent droughts and desiccating winds while the seasonal flooding dominates the rainy season. The water problem was further aggravated by the low water retention capacity of the unique soil group (reddish brown earth) which dominates most part of the dry zone (Mapa et al., 2010). Further, prolonged droughts lower the groundwater table, so that water is inaccessible for wildlife and large scale agriculture.
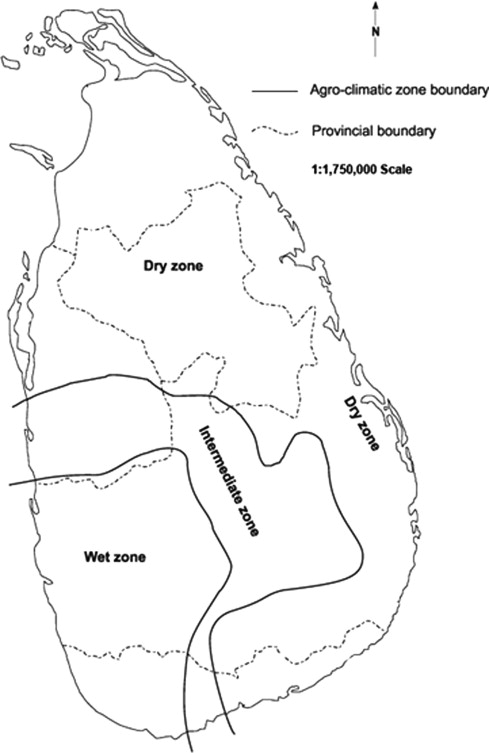
Temporal and spatial disparities in annual rainfall have led ancient farming communities to invent suitable water management systems. The TCS observed today is the outcome their effort to control seasonal flooding and droughts in the dry zone firstly, to ensure the continuous cultivation of paddy during both rainy and dry seasons and secondly to provide a seasonal water supply for domestic and livestock consumption. The undulating topography of the dry zone is well suited for the construction of reservoirs or tanks especially when the underlying basement rock is highly impervious and the overlying weathered rock and soil are usually shallow to moderately deep (Panabokke et al., 2002). The TCS allowed paddy cultivation in low lying arable lands during both rainy and dry seasons.
Ecological implications of the Tank Cascade Systems
The building block of TCSs is a tank (a sub system) which makes up this interwoven irrigation network. Tanks, paddy fields, watersheds and canals are integrated and interwoven with the natural environment (naturalized) (Marambe et al., 2012) which facilitated the evolution of the TCS over two millennia. Villu (a wetland ecosystem in floodplains) are the only natural wetland ecosystems other than rivers in the dry zone (Fig. 4). Both of these TCSs and villus provide many environmental services from its biodiversity and important habitats for endangered Asian elephants (Elephas maximus L.), resident and migrant water birds. Further, a major commonality in these two ecosystems is their plant and animal biodiversity. The major forest ecosystem in the dry zone is tropical dry mixed evergreen forests which represent 54% of the island’s natural forest cover and 16% of its total land area (Gunatilleke et al., 2008). Some common species found in tropical dry mixed evergreen forests and villu are also found in TCSs.
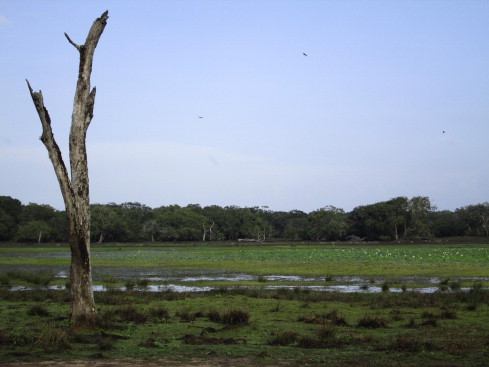
Zones and components of a TCS
Four distinctive zones can be identified in a TCS such as (i) tank bund and tank bed, (ii) associated irrigation channels and paddy fields, (iii) protected forest in the catchment and rainfed uplands and, (iv) gangoda, (hamlet or high elevation household area) (Fig. 5). Each zone had one or several components of ecological significance. Components in the first zone which are unique to TCSs include; sorowwa or bisokotuwa (sluice gate), which is used to release and regulate water being released to paddy fields {three types of sluice gates; goda sorowwa (cistern/valve tower sluice gate), mada sorowwa (mud sluice gate) and keta sorowwa (pie/junction box sluice)}, diyaketa pahana (water level indicator close to the sluice gate), rala panawa (stone liners on the embankment to avoid erosion due to water waves), pita wana, (spills to remove excess water without damaging the tank bund), wew thaulla (upper inundation area; floods during rain and drained off during drought) and mankada {dedicated places for different uses such as bathing (nana mankada) and collection of drinking water (bona diya mankada) and cleaning of animal (bora diya mankada)}, kattakaduwa (interceptor). Components in the second zone include kurulu paluwa (small block of paddy dedicated for foraging birds) and irrigation channels. Gasgommana (tree belt sometimes called athdaduwawa), iswetiya (soil ridge), perahana (filter), landa (shrub lands), chena (rainfed upland cultivation), mukalana (catchment forest), kulu wew (silt trapping small tanks) and, godawala (waterhole) in the third zone and gangoda (hamlet) and thibambe (hamlet buffer) in the fourth zone (Avsadahamy, 2003; Dharmasena, 1994, 2010). Each component has a unique role in maintaining TCSs and their sustainability are detailed below.
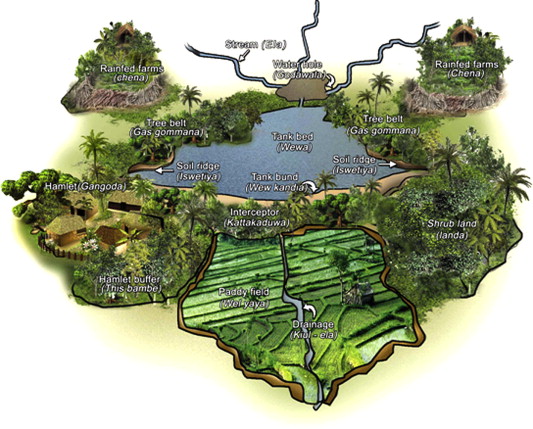
Interceptor (Kattakaduwa)
This is the stretch between tank bund and paddy fields which is technically similar to the width of base of the tank bund. The main function of the interceptor is absorbance of salts and heavy metals from seepage water across the embankment (referred in modern science as phyto-remediation). It also acts as a downstream wind barrier and helps to protect soil from erosion as majority of species consists with well developed root systems. Interceptors contain a range of naturalized flora and fauna in the downstream side of the earth embankment of the tank. Tree species such as Terminalia arjuna (Roxb.) Wight & Arn and Strychnos potatorum L.f., which act as purifying agents of water via their roots and seeds respectively, were found in locations dedicated for drinking water (bona diya mankada). Other common species in the area are Madhuca longifolia (Konig) Macbr., Derris spp. and Mangifera indica L. Dharmasena (2010) found that around 77 species occurs in interceptors of which 13 species are unique to this naturalized habitat. Microhabitats were therefore created for numerous fauna (Dharmasena, 2010). The tree species present here were useful for cottage industries, medicines, firewood, fodder and food for the farming communities.
Tree belt (Gasgommana) and water filter (Perahana)
Tree belt is the large size tree belt located in upper inundation area along the tank which acts as a wind barrier and reduces the occurrence of waves in the tank. Further, due to this reason, it reduces evapotranspiration. In addition, during high flood period, this area provides different habitats for fish and other aquatic species. Water filter which is dominated by sedges, ratten, shrubs and grasses retains sediments in runoff water between tank and tree belt and the main function is purification of water.
Catchment forest (Mukalana)
The forest cover in the catchment was community owned and was protected by controlling felling trees and clearing for agricultural expansion (Hitinayake et al., 2008). The environmental services provided by the forest were valued in farming communities and used only for the sustainable extraction of some non timber forest products such as medicinal herbs for ayurvedic preparations (a branch of medicine mainly in India and Sri Lanka). However, the key role of the forest was to increase the groundwater table through infiltration and gradual release of water to the tank during the dry season (Hitinayake et al., 2008). Another principle of TCS is the ecological harmony of land use where the rich alluvial humic gley soils were used for paddy fields, while the sloping areas of reddish brown earth soils in the upper segments of the catena, were devoted to shifting cultivation that allowed the soils to recuperate after several years of fallow. The most infertile areas of the watersheds were left intact under rocks and forests, except for being used for spiritual purposes (Madduma Bandara, 1995).
Check dams (Potawetiya) and soil ridges (Iswetiya)
Check dams were used to retain incoming sediments from the upper catchment. These check dams reduced the velocity of water and once it is naturalized resembled to marshy land with numerous semi aquatic fauna and flora. In addition, check dams had stored excess water in the upper catchment before gradually releasing to the tank during the dry season. The soil ridge reduces the soil erosion by blocking sediments and hence siltation in the tank.
Silt trapping small tanks (Kuluwew) and waterholes (Godawala)
Silt-trapping small tanks have been constructed inside the catchment forest. The water in these small tanks were not used for agricultural purposes but had a fundamental role to trap silt and to regulate the water flow to the main tank. In fact, these tanks were structurally simple usually surrounded by the forest and had no sluice gate. The groundwater table, subsurface water flow and river base flow were augmented using these tanks which were further useful a source of water for the forest vegetations and wildlife (Avsadahamy, 2003). Waterholes were located at the inlet of the tank and mainly acted as a drinking water source for wildlife and livestock. In addition waterholes provided benefits such as sedimentation, slat filtration and conservation of macro and micro aquatic fauna. During strong storm events waterholes buffered water level in the downstream, this reduces the risk of breaking the embankment. Waterholes were common in all tanks but in contrast silt trapping small tanks were only found in large tanks (Avsadahamy, 2003).
Other essential components
The dedicated area for foraging birds (kurulu paluwa) is a strip of cultivated paddy land adjacent to a tank bund or at the downstream end of the paddy fields abutting the next tank, is primarily dedicated to birds. Although its exact function in the past is unclear, the land was left without harvesting to allow birds to feed on, thus minimizing avian crop damages. The upper inundation area is also act as a common grazing ground for livestock animals and wildlife. The dung accumulated not only nourishes the ground for continuous supply of grasses but also tank, thus provide nutrient for aquatic flora and fauna. Shrub lands (landa) also act as a buffer among paddy fields, homegardens and, forests keeping minimum wild animal damages to crops and householders.
These tanks were comprised of several other hydraulic engineering elements such as the pie/junction box sluice and tower sluice/valve pit (Figs. 6 and 7). The pie/junction box valve pit were used mainly in small scale tanks to release the water from the tank’s surface. Using this method, salts were trapped in the tank and paddy fields were protected from salinization. Parker (1907) stated that “since about the middle of last century, open wells, called ‘valve-towers’ when they stand clear of the embankment and ‘valve-pits’ when they are in it, have been built at numerous reservoirs in Europe. Their duty is to hold the valves, and the lifting-gear for working them, by means of which the outward flow of the water is regulated or totally stopped. Such also was the function of the valve-pit of the ancient Sri Lankan engineers; they were the first inventors of the valve-pit, more than 2100 years ago”.
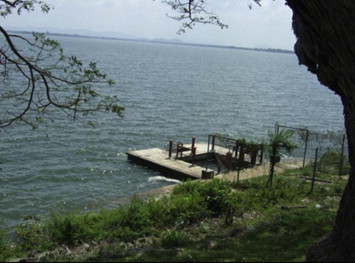
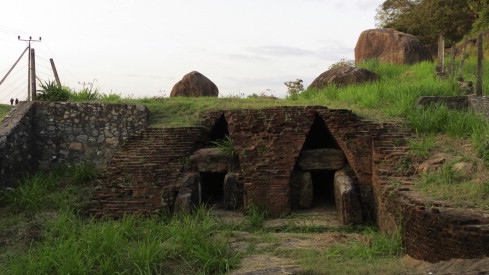
The channel technology is unique to ancient Sri Lanka. As the feeder channels had only one embankment which was constructed along contour lines, the channels were also able to store some amount of water. This reduced the devastating effects of storm water during the rainy season and supported the agriculture and wildlife alongside (Avsadahamy, 2003). All components of the TCSs are man-made, firstly to provide water for human consumption and secondly, support the existence of local biodiversity and its ecosystem services. With further advancement of irrigation technology, perennial rivers were also diverted to large scale tanks (Fig. 8).
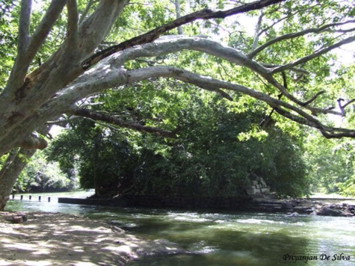
Management of Tank Cascade Systems
The governance of TCS was mainly based at the village level and therefore all components in tanks have been progressed with the socioeconomic harmony of villagers (Avsadahamy, 2003). Analysis of TCSs shows that their management was largely done by the social system with established institutions and leadership structures with cultural and spiritual norms that respected life in its all forms (Dharmasena, 2004; Tennakoon, 1986). Early practitioners adhered firstly, to have an adequate volume of water in every tank in a cascading valley even in a year of below-average rainfall and secondly, instituting a regulated flow of water from one tank to another downstream thereby, avoiding a sudden influx of large volumes of water (Tennakoon, 2005). By doing so the risk to tank bunds breaking was minimized.
Only sketchy and rudimentary evidence exists from ancient times indicating the ownership, maintenance obligations, sanctions, penalties and taxation in TCSs (Madduma Bandara, 1995). However records shows that the velvidane was the officer in charge for the management of TCSs. In the pre-colonial era, TCSs were managed through an ancient custom of compulsory labor. But, this system was abolished by the British in 1832 AD because it was considered a form of slavery (Panabokke et al., 2002). An alternative maintenance scheme for the compulsory labor was not introduced which resulted in gradual abandonment of TCSs (Madduma Bandara, 1985). This contrast with previous management where farmers did not have tenure to TCS, tanks were community owned and were properly maintained. The farmer’s willingness to sustain the system was also declined over several centuries (Madduma Bandara, 1985). In the post-independence era, however, large scale development projects to manage water have been undertaken in the dry zone; the TCS is now managed by both the government and farmer organizations.
Cultural diversity of Tank Cascade Systems
The primary function of a village tank was to irrigate dry lowland plains for paddy farming during major cultivation seasons. Water was an essential resource for paddy farming in the dry zone but limited both temporally and spatially although soil, other climatic factors and soil topography are ideal for agriculture. Hence, rural dry zone communities time to time developed TCSs with limited equipments and local knowledge. In this case, selection of land for establishment of tank and other components of the system were based on ecological principles. There is therefore a close association between paddy cultivation and the evolution of TCSs. Although not properly documented, many rituals and cultivation systems as adaptations to droughts have evolved within TCSs. For instance, Bethma farming is a method of paddy faming which involve the conservation of tank water during droughts using agreed rules for sharing water in scarce situations. In this case the village leader, an experienced farmer, defines the cultivable area of paddy during the drought, using a measuring scale called diyaketa pahana. The cultivable area is in close proximity to main irrigation channels. Farmers are given proportionally similar sized blocks as those cultivate during the rainy season. This helps to conserve the available irrigation water by reducing to minimize conveyance losses to the fields away from irrigation channels which cultivate during the rainy season (Dalupotha, 2002). This system reduced the vulnerability of the crop by cultivating an area which can only be irrigated using the available water in the tank during the drought. If the drought continued to exist, Madathawalu farming (farming in the tank bed) was recommended to preserve the seed paddy for the next season (Dalupotha, 2002). However, Thiruchelvum (2005) found that stronger farmer organizations were more efficient in productivity and technical efficiency of paddy farming than weaker ones and suggested that strengthening farmer organizations would increase agricultural production efficiency under the bethma system.
Customs adapted by local communities to manage water resources have been inherited over many generations. For example kem systems (a form of crop protection measures), rituals, chanting based on religion, and starting activities based on an auspicious times related to the movement of moon (Marambe et al., 2012) are still in use. Folk poetries were very common during all the field activities of paddy farming were not necessarily limited to field preparation, weeding and harvesting. These folk poetries are well documented locally; they provide insights for research in traditional ecological knowledge because they are encapsulated with the knowledge and experience.
Conclusions: historic and modern challenges
Multiple invasions from South India into Sri Lanka in 437–367 BC have been destructive for the survivability the TCSs. Thereafter, during the colonial era, TCSs were neglected and abandoned. Because the TCS was the backbone of traditional economy, the destruction of this system had far-reaching effects. Although the TCSs did not completely collapse in the ancient times, they were temporary declined due to malaria epidemics and the political instability. However, because modern-day engineers believed that centralized large-scale irrigation schemes are more efficient than the decentralized TCSs, were replaced by large scale reservoirs and high capacity feeder channels. These large scale feeder channels now have the capacity to control water flow to remaining TCSs which are directly linked with large reservoirs generating hydropower. Panabokke et al. (2002) recommended that hydrology of an entire cascade needs to be assessed and understood in order to make the best use of available water resources within the cascade. It is essential to recognize the knowledge on hydrology of an entire cascade before any intervention to any individual tank in the cascade is contemplated. However, many large diameter agricultural wells (agro-wells) are being excavated as a solution for increasing demand for water (Panabokke et al., 2002). This drastically reduces the groundwater table and degrades associated ecosystems because the density of small tanks and feeder channels is reduced due to multipurpose irrigation projects and human settlements.
The TCS has been successful over two millennia in sustaining a variety of ecosystems in the dry zone of Sri Lanka. Many lessons can be adopted from TCSs to ensure the present tank system is sustainable. However, ad hoc raising of dams and spillways and just desilting of tank in the recent past has seriously disrupted the hydrological balance in TCSs (Panabokke et al., 2002). A noticeable increase of population has also unbalanced subsistence farming methods and aggravated multiple market forces and exchange of outside inputs (Panabokke et al., 2002).
It is not now feasible to adopt the same land use which was in traditional TCSs. The challenge is to bring some elementary changes in planning, designing, and managing TCS to sustain the ecosystem services provided. Importantly, we need to understand the underlying principles and mechanisms of the evolution of TCSs. Then, treating this as a system, while understanding the role of each component with perhaps, modification of some of the roles with modern technologies with planning of entire agriculture and livelihood as a unit, as in the past, is a must to bring the value of TCSs to the next generation. It is important to note here that only the system will sustain not the components, hence collective tank based sharing system should be strengthen with modern techniques and technologies without damaging them.
Acknowledgements
Authors acknowledge the financial support provided by the British Ecological Society, United Kingdom and the National Science Foundation of Sri Lanka to present this paper at the 11th Quadrennial Meeting of the International Association for Ecology (INTECOL 2013), and also those unanimously provided constructive comments on the manuscript.
References
- Avsadahamy, 2003 U.B. Avsadahamy Wewa (in Sinhala). (first ed.), Siri Printers, Hingurakgoda, Sri Lanka (2003)
- Brohier, 1935 R.L. Brohier, (Translated by Piyasena L.)Ancient irrigation work of Ceylon (Sri Lanka). (first ed.), Mahaweli Center, Mahaweli Authority of Sri Lanka, Colombo (1935)
- CBSL, 2012 CBSL Annual Report. Sri Lanka, Central Bank of Sri Lanka, Colombo (2012)
- Dalupotha, 2002 M.K. Dalupotha Tank and Culture (in Sinhala). S. Godage & Brothers, Colombo, Sri Lanka (2002)
- Dharmasena, 1994 P.B. Dharmasena Conservation farming practices for small reservoir watersheds: a case study from Sri Lanka. Agroforestry Systems, 28 (1994), pp. 203-212
- Dharmasena, 2004 P.B. Dharmasena Small tank heritage and current problems. Village tank categorization. M.M. Aheeyar (Ed.), Small Tank Settlements in Sri Lanka, Kobbakaduwa Agrarian Research and Training Institute, Colombo, Sri Lanka (2004)
- Dharmasena, 2010 Dharmasena, P.B., 2010. Essential components of traditional village tank systems. In: Proceedings of the National Conference on Cascade Irrigation Systems for Rural Sustainability. Central Environmental Authority, Sri Lanka, 9 December 2010.
- Geeganage, 2013 Geeganage, A., 2013. The village way of experiencing Vesak around Maduru oya. <http://trips.lakdasun.org/the-village-way-of-experiencing-vesak-around-maduru-oya.htm> (accessed on 16.11.2013).
- Gunatilleke et al., 2008 N. Gunatilleke, R. Pethiyagoda, S. Gunatilleke Biodiversity of Sri Lanka. J. Natl. Sci. Foundation Sri Lanka, 36 (2008), pp. 25-62
- Hitinayake et al., 2008 G. Hitinayake, D. Silva, I. Vithanage Ecological Farming Systems and Technologies (Sinhala Version). Department of Crop Science, Faculty of Agriculture, University of Peradeniya, Peradeniya, Sri Lanka (2008)
- Madduma Bandara, 1985 C.M. Madduma Bandara Catchment ecosystems and village tank cascades in the dry zone of Sri Lanka: a time-tested system of land and water management. J. Lundqvist, et al. (Eds.), Strategies for River Basin Management, Linkoping, Sweden (1985)
- Madduma Bandara, 1995 C.M. Madduma Bandara Tank cascade systems in Sri Lanka: Some thoughts on their development implications. K.A. Haq, C.M. Wijayaratne, B.M.S. Samarasekera (Eds.), Summaries of papers presented at Irrigation Research Management Unit seminar series during 1994, Colombo, Sri Lanka, IIMI (1995), p. 14
- Mapa et al., 2010 R.B. Mapa, S. Somasiri, A.R. Dassanayake Soils of the Dry Zone of Sri Lanka; Morphology Characterization and Classification. Soil Science Society of Sri Lanka, Peradeniya, Sri Lanka (2010)
- Marambe et al., 2012 B. Marambe, G. Pushpakumara, P. Silva Biodiversity and agrobiodiversity in Sri Lanka: village tank systems. S.I. Nakano, T. Yahara, T. Nakashizuka, S. Yamamoto (Eds.), Biodiversity Observation Network in the Asia-Pacific Region, Springer, Japan (2012), pp. 403-430
- Mendis, 2003 Mendis, D.L.O., 2003. Environment and conflict: a Kuhnian paradigm-based approach to understanding some socio-cultural and economic causes of problems and conflicts in modern irrigation projects in southern Sri Lanka. In: Water for People and Nature. Ministry of Irrigation and Water Management, Sri Lanka, pp. 95–122.
- Panabokke et al., 2002 C.R. Panabokke, R. Sakthivadivel, A.D. Weerasinghe Evolution, Present Status and Issues Concerning Small Tank Systems in Sri Lanka. International Water Management Institute, Colombo, Sri Lanka (2002)
- Parker, 1907 H. Parker Ancient Ceylon. Lucas, London, United Kingdom (1907)
- Punyawardane, 2008 B.V.R. Punyawardane Rainfall in Sri Lanka and Agro-ecological Zones (Sinhala Version). Department of Agriculture Press, Peradeniya, Sri Lanka (2008)
- Tennakoon, 1986 M.U.A. Tennakoon Drought hazards and rural development, Central Bank of Ceylon (Sri Lanka). Colombo, Sri Lanka (1986)
- Tennakoon, 2005 M.U.A. Tennakoon Ellangawa (Sinhala Version). Godage and Sons, Colombo, Sri Lanka (2005)
- Thiruchelvum, 2005 S. Thiruchelvum Agricultural production efficiency of Bethma cultivation in Mahaweli system H. Sri Lankan J Agric. Econ., 7 (2005), pp. 1-20
- UNCCD, 2005 UNCCD Revitalizing Traditional Knowledge a Compilation of Documents and Reports from 1997–2003. United Nations Convention to Combat Desertification, Bonn, Germany (2005)