Distribution of Freshwater Plant Communities and Halophytes in Coastal Region, Muan-gun, South Korea
Abstract
The distribution of freshwater plant communities and the relationships between them and sand dunes, salt marshes, and reclaimed lands of the southwestern coasts of South Korea was studied along with plant factors. Competitor (C), stress tolerator (S), and ruderal (R) (CSR) ecological strategies were also evaluated. To elucidate the relative significance of competition, stress, and disturbance (CSR) in the distribution process of plant communities, the CSR distribution model was adopted. Many coastal sand dune plants (12 species) showed CR/CSR strategies. In salt marshes, nine species showed CR strategies. In reclaimed land, 16 of the 19 species were allocated to factors related to ruderal strategies such as R/CR and CR as well as R/CSR, SR/CSR, CR/CSR, and SC strategies. The most abundant species in freshwater wetlands were Ceratophyllum demersum and six other species, which all exhibited R/CR strategies. The above four habitat type species were categorized using CSR classification. The strategies were different between water plants. From first to next water plant stages progression of early succession appeared in studied aquatic wetlands. Seawater-related plant species had similar C-SR-R strategies related to sand dunes, salt marshes, and reclaimed wetlands and R/CR strategies in freshwater wetlands. Two dominant succession species, Nelumbo nucifera and Phragmites communis, showed C/SC or SC strategies. CSR triangles may provide a useful scientific basis for protecting and restoring coastal wetlands and by reducing atmospheric CO2, these resources help stabilize global temperatures.
Keywords
sand dune, salt marsh, reclaimed land, freshwater wetland, CSR strategy, plant distribution
Introduction
The patterns and relationships between plant, soil, and water data from freshwater wetlands, coastal sand dunes, salt marshes, and reclaimed lands may be derived from the field or laboratory, designed experiments or surveys, and nonlinear relationships (Costa et al., 1996; Lee et al., 2020; Son et al., 2020; Ter Braak, 1986; Ter Braak and Smilauer, 2018). In the distribution of the four habitats, the chemo-physical factors are salinity, flooding and frequency, soil texture, groundwater levels, climate, altitude, topography, soil texture, Ca2+, Mg2+, K+, organic matter (OM), pH, and cation exchange capacity. The biological factors are competition, invasion, stress or disturbance tolerance, and grazing (Alvarez-Rogel et al., 2007; Lee and Kim, 2018; Lee et al., 2020; Myeong, 2010).
Because of the transitional situation between the sea and terrestrial ecosystems, the southwestern coastal wetlands of South Korea include various habitats, such as salt marshes, salt swamps, and sand dunes (Bertness and Ellison, 1987; Elmas, 2017; Hong et al., 2010; Gu et al., 2018; Ihm et al., 2007; Lee and Kim, 2018; Son et al., 2020). In general, this seems to be because long-term permanent changes in tidal flow patterns affect vegetation as well as the chemical and physical factors of the soil. The frequency of flooding is more important than elevation in predicting marsh plant zones. The studies done to date indicated that redox potential, ionic composition, the moisture content of soil, latitude, and topographical, and climatic factors may play some roles in vegetation distribution (Kim, 2005; Martinez et al., 2008; Pomeroy and Wiegert, 1981; Silander and Antonovics, 1982; Yang et al., 2021).
Grime (1974) postulated that the typical species in three environmental extremes possess distinct features: competition (C), stress tolerance (S), and ruderality (R). Species positions can be plotted in a triangular diagram to indicate their relative importance. The interactions between the three basic selective pressures considered in the competitor, stress tolerator, and ruderal (CSR) model theory of coastal plants are competition (low stress and low disturbance habitats); stress tolerance (high stress and low disturbance habitats); and ruderality, which occurs in low stress and high disturbance habitats (Ciccarelli, 2015; Elmas, 2017; Lee et al., 2020; Myeong, 2010). In a multivariate analysis, Grime (2002) and Pierce et al. (2007) reported that a CR strategy occurred in habitats disturbed by ruderality (a plant's ability to survive in disturbed conditions) and competition. The percentage of ruderalism reflects the dominant disturbance intensity in different habitats. Coastal plant strategies can change from CR to C (from competitor-ruderal to competitor). Competition also affects the niche differentiation of plant species. More intense disturbances increase functional diversity and species diversity and the inhabiting dominant stress-tolerator plants. CR plants require rich soil nutrients and, as perennial, biennial, or annual plants, can adapt and survive in coastal habitats. The dynamics of the changes in water properties in freshwater- and seawater-related habitats due to disturbances have differed over time (Alahuhta et al., 2011; Bornette and Puijalon, 2011; Ihm et al., 2007; Lee and Kim, 2018; Rooney and Kalff, 2000). It was shown that one of the reasons for ruderal competitor success was that chemical properties, such as pH, OM contents, and nitrates, are important determinants of plant ecology and distribution.
Sea reclamation provides land for agricultural production, urban and industrial development, and port construction (Cho et al., 2018; Hong et al., 2010; Min and Kim, 2000; Suzuki, 2003). Currently, the development of coastal zones that started in the 1950s, has entered a peak period, especially in developing countries. Notably, the scale and scope of reclamation in coastal lands are larger than in interior lands and pose substantial environmental risks. Tidal flats on coasts in Eastern Asia are being converted to sea-reclaimed land (Min and Kim 1999, 2000; Hong et al., 2010). The reclaimed land has characteristics of both coastal soils and inland salt marshes (Min and Kim, 1999; Yang et al., 2021). After reclamation, the sediment becomes the common soil as soil salts leach over time. The leaching rate is influenced by rain intensity, topography, geographical properties, and soil texture. Therefore, plant species growing in reclaimed lands differ in their inherent salt tolerance (Ihm and Lee, 1995; Min and Kim 2000; Yang et al., 2021; Zhu et al., 2014), and vegetative zonation develops according to the topography or distance from the high water mark to sites farther inland. In areas that are periodically inundated by tides, the zonation of the plant community is not regarded as successional because the tidal-flat vegetation in these areas is not formed by interactions among plants but by erosion and silt accumulation (Castillo and Moreno-Casasola, 1996; Min and Kim 2000; Yang et al., 2021). On reclaimed lands, plant succession proceeds after reclamation (Min and Kim, 1999). The main factor that determines succession on reclaimed lands is the soil environment and, because in these areas the soil properties change rapidly, the rate of vegetational succession is faster on reclaimed land than on common land. In the early stages of succession, the plant species are mostly annuals. Species diversity reaches a maximum 10 years after reclamation. The variability in succession rates is related to the pattern of creek distribution along different topological altitudes (Cho et al., 2017; Kim et al., 2011; Min and Kim, 2000; Yang et al., 2021).
The objectives of this study were as follows. The significant differences in plant traits among plant communities in freshwater ecosystems near the coasts and in coastal ecosystems (Lee et al., 2020; Pierce et al., 2007; Son et al. 2020; Yang et al. 2021) were evaluated. It was expected that within the habitats (coastal sand dunes, salt marshes, reclaimed lands, and freshwater ecosystems), plants could be classified by CSR variables. It was also expected that this method would demonstrate the relative importance of plant species succession strategies in coastal seawater and freshwater environments.
Methods
Five wetlands, seven plant factors, and six water factors were examined and the vascular plant distributions were analyzed in freshwater wetlands in Muan peninsula of South Korea (Figure 1).
Study Area
Eighteen freshwater wetland plant communities were sampled from the Muan peninsula on the west coast of South Korea (Figure 1). We evaluated five freshwater wetlands. The habitat disturbance and status of the study sites are included in Table 1.
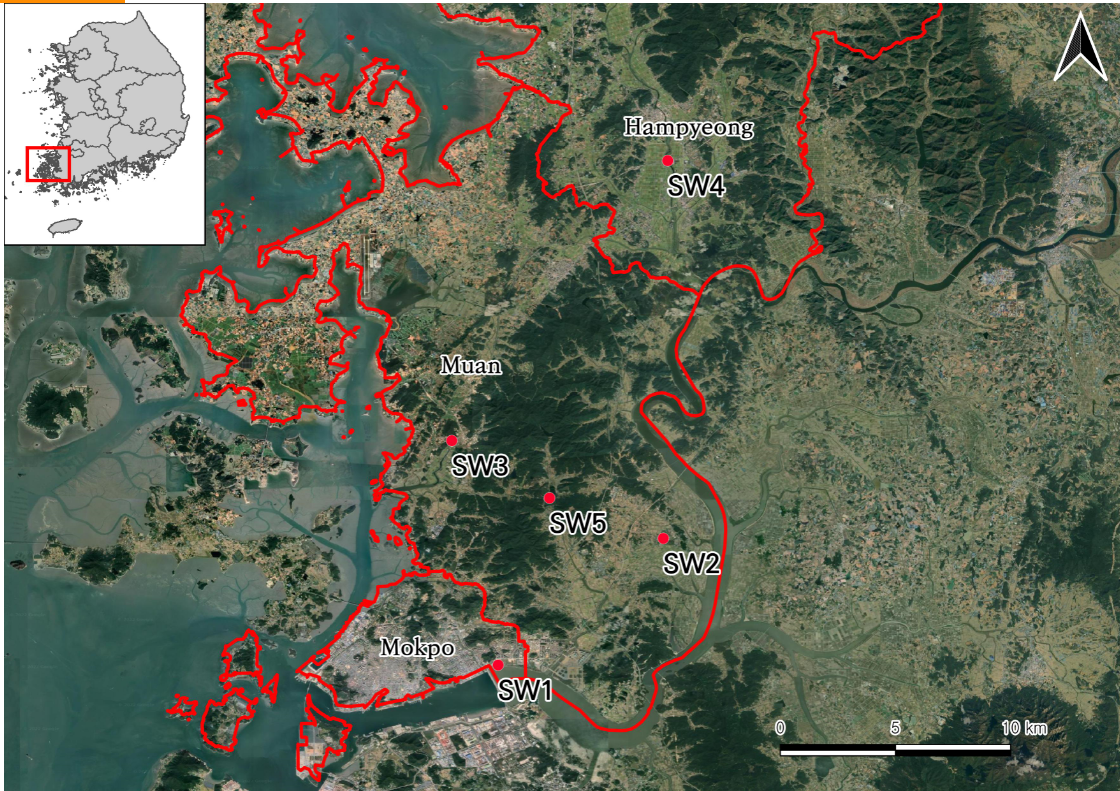
Abbreviation | Local Name | Latitude | Longitude | Type of aquatic plants | Dominant community |
---|---|---|---|---|---|
SW1 | Ogam riverside ecological park | 34.802346 | 126.446115 | Emerged plant | Phragmites communis |
SW2 | Muan Hoesan white lotus pond | 34.862366 | 126.524458 | Floating-leaved plant | Nelumbo nucifera |
SW3 | Cheonggye-myeon Yonggyecheon | 34.908677 | 126.424156 | Submerged plant | Ceratophyllum demersum |
SW4 | Hampyung Hwayang-ri Hampyungcheon | 35.041393 | 126.526551 | Submerged plant | Oenanthe javanica |
SW5 | Gamdon reservoir | 34.881523 | 126.470487 | Emerged plant | Typha orientalis |
Plant research by species
We performed canonical correspondence analysis (CCA) and evaluated competitor (C), stress tolerator (S), and ruderal (R) (CSR) ecological strategies in the 18 communities (1 x 1 m2 quadrants, three replicates per species) from five freshwater wetland areas from July-August 2021 (Table 1).
The five areas and their dominant species as shown in Table 1 include Ogam riverside ecological park, Phragmites communis (common reed); Muan Hoesan, white lotus pond, Nelumbo nucifera (lotus flower); Yonggyecheon, Ceratophyllum demersum (Coon’s tail); Hampyungcheon (Figure 2), Oenanthe javanica (Java water-dropwort); and Gamdon reservoir, Typha orientalis (Oriental cattail).
Plant factor analysis
We analyzed several plant factors (Hodgson et al. 1999; Lee et al. 2020; Babur et al. 2021; Pierce et al., 2013) (Table 2). The coverage (%), canopy height (CH, mm), lateral spread (LS, mm), leaf area (LA, mm2), leaf dry weight (LDW, mg), leaf dry weight/water-saturated fresh mass (LDMC, %), specific leaf area (SLA, mm2mg-1), flowering period (FP), and flowering start (FS) were measured. We selected a total of 18 shrub and herbaceous species for the analysis of plant functional factors.
Canopy height (six-point model) | Leaf dry matter content (%) | Flowering period (# of months) | Lateral spread (six-point model) | Leaf dry weight (mg) | Specific leaf area (mm2 mg-1) | Flowering start (month) | |
---|---|---|---|---|---|---|---|
Means | 4.3 | 25.1 | 2.6 | 5.1 | 423.8 | 24.29 | 5.2 |
SD | 0.5 | 17.3 | 0.6 | 1.5 | 1011.7 | 20.12 | 0.9 |
N | 18 | 18 | 18 | 18 | 18 | 18 | 18 |
MAX | 6 | 83.3 | 4 | 6 | 6728.0 | 76.57 | 6 |
MIN | 1 | 7.7 | 2 | 1 | 5.0 | 0.22 | 3 |
Range | 5 | 75.6 | 2 | 5 | 6723.0 | 76.35 | 3 |
Water analysis
We examined the water environment near the plant leaves. Water samples (three replicates per species) were used for physicochemical analysis (Lee et al., 2020) (Table 3). Salinity, electrical conductivity, nitrate-N, ammonium-N, phosphate, and pH were determined.
Salinity (PSU) | EC (μS/cm) | Nitrate-N (mg/L) | Ammonium-N (mg/L) | Phosphate (mg/L) | pH | |
---|---|---|---|---|---|---|
Means | 0.179 | 378 | 0.6 | 0.45 | 0.161 | 6.70 |
SD | 0.122 | 243 | 0.8 | 0.26 | 0.209 | 0.99 |
N | 18 | 18 | 18 | 18 | 18 | 18 |
MAX | 0.513 | 1042 | 4.7 | 0.36 | 1.145 | 9.78 |
MIN | 0.069 | 14.7 | 0 | 0.15 | 0 | 6.22 |
Range | 0.444 | 895 | 4.7 | 1.21 | 1.145 | 3.55 |
Statistical analyses
We conducted a CCA analysis of water and plant factors using CANOCO 5.1 (Lee et al. 2020; Ter Braak and Smilauer 2018) (Figure 3 and 4). The CSR strategies were formulated using plant functional factors and triangular diagrams were generated according to Hodgson et al. (1999) and Pierce et al. (2013) using Sigma Plot 13 (www.sigmaplot.com).
Results
The most abundant species (Table 4 and Figure 3-5) in the freshwater wetlands were Ceratophyllum demersum, Hydrilla verticillata, Lemma paucicostata, Myriophyllum erticillatum, Najas marina, Persicaria longiseta, and Persicaria thunbergii (coverage = 100%). The second most abundant species was Trapa japonica (coverage = 90%), which exhibited R/CR strategy, and the third most abundant species were Phragmites communis and Zizania latifolia (coverage = 80%), which exhibited SC and R/CR strategies.
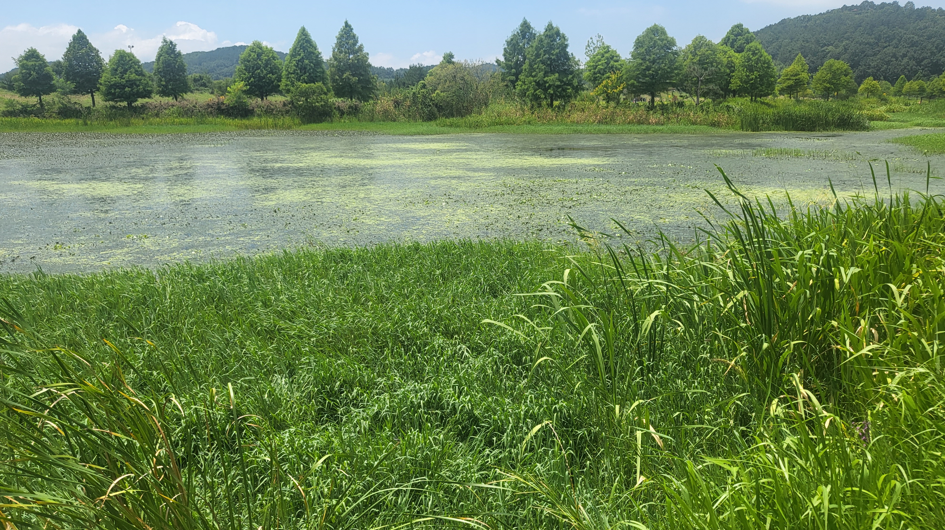
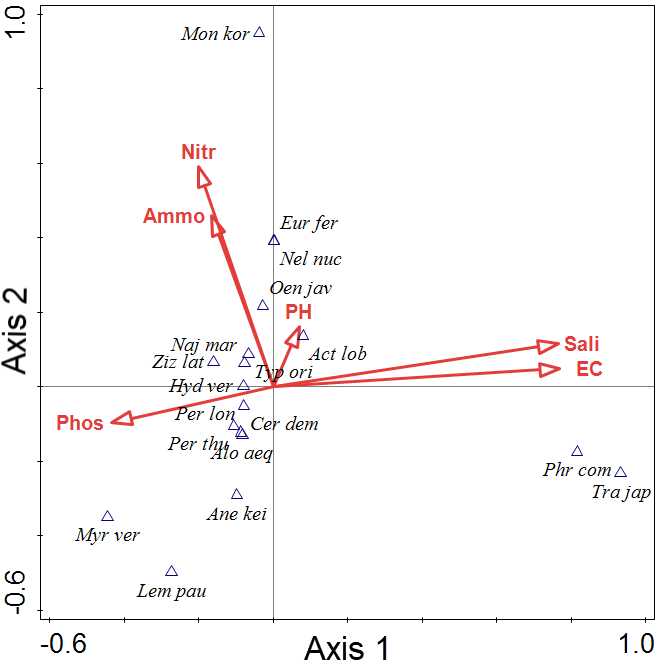
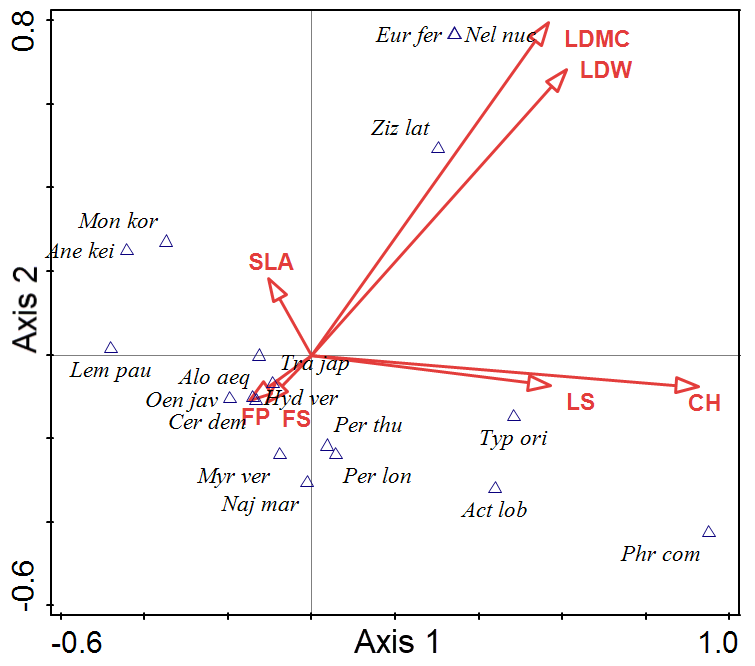
Species | Percentage from Caccianiga et al.(2006) | Strategy type | Plant cover (%) | ||
---|---|---|---|---|---|
C (%) | S (%) | R (%) | |||
Actinostemma lobatum | 39.0 | 0.0 | 61.0 | R/CR | 40 |
Alopecurus aequalis var. amurensis | 33.5 | 0.0 | 66.5 | R/CR | 60 |
Aneilema keisak | 29.2 | 0.0 | 70.8 | R/CR | 60 |
Ceratophyllum demersum | 38.8 | 0.0 | 61.2 | R/CR | 100 |
Euryale ferox | 46.4 | 53.6 | 0.0 | SC | 30 |
Hydrilla verticillata | 37.6 | 0.0 | 62.4 | R/CR | 100 |
Lemma paucicostata | 9.3 | 0.0 | 90.7 | R | 100 |
Monochoria korsakowi | 24.3 | 0.0 | 76.6 | R/CR | 90 |
Myriophyllum verticillatum | 27.0 | 0.0 | 73.0 | R/CR | 100 |
Najas marina | 38.9 | 0.0 | 61.1 | R/CR | 100 |
Nelumbo nucifera | 73.4 | 26.6 | 0.0 | C/SC | 100 |
Oenanthe javanica | 25.8 | 0.0 | 74.2 | R/CR | 70 |
Persicaria longiseta | 38.2 | 0.0 | 61.8 | R/CR | 100 |
Persicaria thunbergii | 35.0 | 0.0 | 65.0 | R/CR | 100 |
Phragmites communis | 52.0 | 48.0 | 0.0 | SC | 70 |
Trapa japonica | 34.4 | 0.0 | 65.6 | R/CR | 90 |
Typha orientalis | 58.9 | 0.0 | 41.1 | CR | 20 |
Zizania latifolia | 73.9 | 0.0 | 26.1 | R/CR | 80 |
Thirteen of the 18 species were allocated to factors related to R and C strategies (Table 4, Figs. 4 and 5). They displayed adaptative strategies reflecting the various environments (Lee et al., 2020; Yang et al., 2021). The 12 species with R/CR strategies were Actinostemma lobatum, Alopecurus aequalis var. amurensis, Aneilema keisak, Ceratophyllum demersum, Hydrilla verticillata, Monochoria korsakowi, Myriophyllum verticillatum, Najas marina, Oenanthe javanica, Persicaria longiseta, Persicaria thunbergii, and Trapa japonica. The two species displaying C/SC and SC strategies were Nelumbo nucifera and Phragmites communis.
The three species with CR, R/CR and SC strategies were Typha orientalis, Zizania latifolia, and Euryale ferox (Table 4 and Figure 4). The canopy height was 5mm-100cm in R species, 100–300 in C species and 90cm in S species. The LDMC was 0.004-10.606, 12.894-40.233 and 48.776% and the SLA was 0.7-70.6, 9.8-17.9 and 34.7 mm2/mg, respectively.
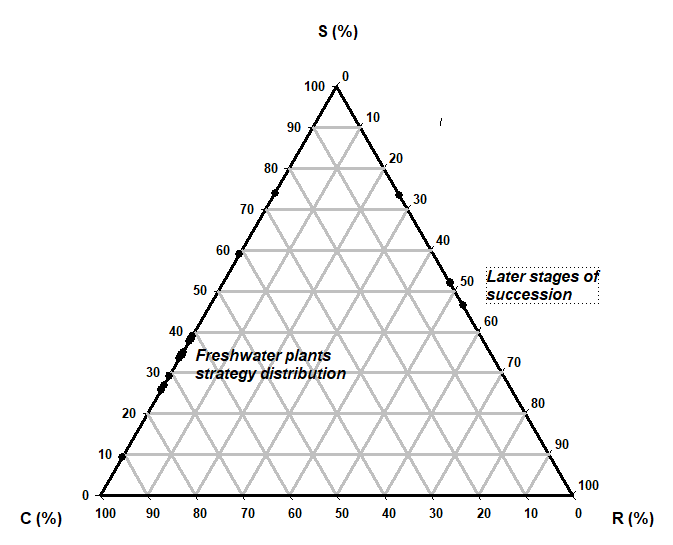
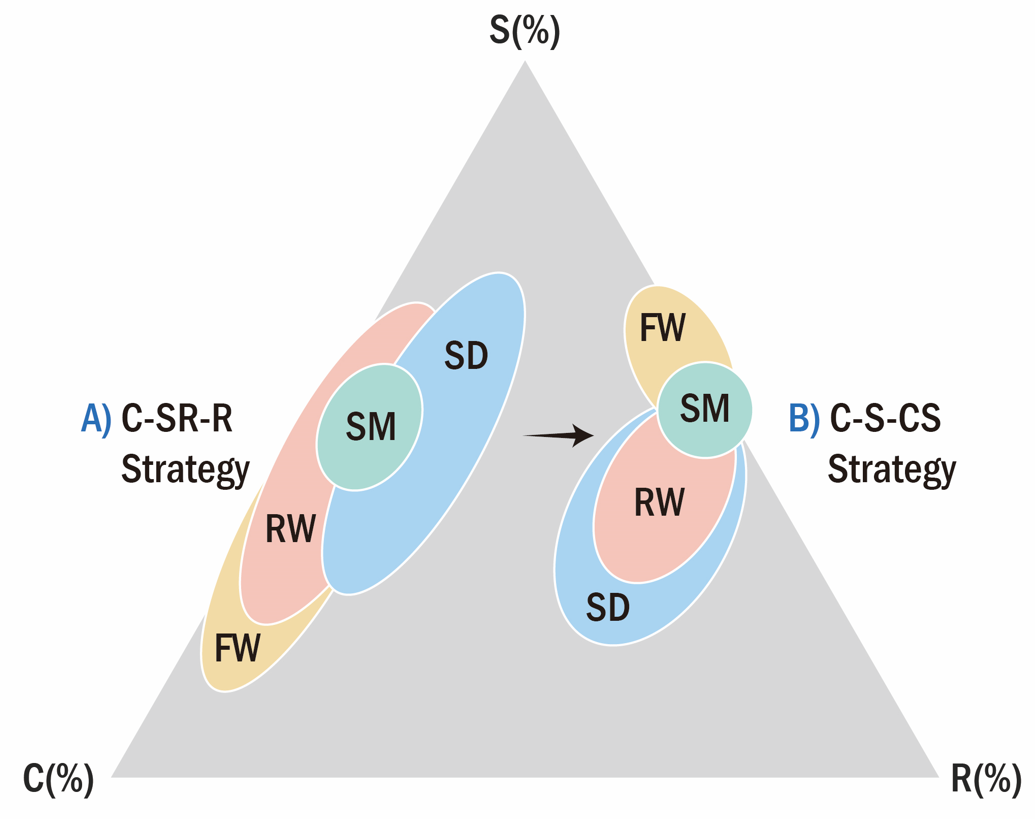
Figure 3 shows the CCA bi-plot diagram of the freshwater wetlands on the Muan peninsula of South Korea (Lee et al. 2018, 2020). Axes 1 and 2 are the vegetation plots and the water factors (Table 3). The water factors are shown as arrows. The 18 plant communities, classified into three groups, are displayed on axes 1 and 2. Group 1 was correlated with salinity and electrical conductivity, group 2 was correlated with nitrate-N and ammonium-N, and group 3 was correlated with phosphate content. The explanatory variables accounted for 35.18% of the variation. The eigenvalues of axes 1 and 2 were 1.0000 and 1.0000, respectively. The lengths and positions of the arrows provide information about the relationship between the water factors and the derived axes (Figure 3). Arrows that are parallel to an axis (e.g., salinity and axis 1) indicate a correlation, and the length of the arrow indicates the strength of the correlation. Thus, EC and salinity are related to axis 1. Consider Monochoria korsakowi, with a high score on axis 2. It is associated with nitrate-N and ammonium-N, and the correlation between them and axis 2 is positive (shown by the direction of the arrows). Myriophyllum verticillatum and Lemna paucicostata are related to axis 1, while their correlations are negative.
Figure 4 presents the CCA ordination results of the coastal plant dataset. The 18 community scores are plotted along axes 1 and 2 (Table 3). The CCA eigenvalues for the first two ordination axes were 1.0000 and 0.9999, respectively. The explanatory variables accounted for 41.13% of the variance in the community data. LDMC and LDW were related to both axis 1 and 2. Euryale ferox and Nelumbo nucifera had high scores on both axis 1 and 2. Axis 1 was associated with LS and CH. Phragmites communis and Typha orientalis had a high score on axis 1.
![]() |
![]() |
a) Imperata cylindrica var. koenigii community (SC/CSR) in sand dune | b) Carex scabrifolia community (SC) in salt marsh |
![]() |
![]() |
c) Aeschnomene indica community (RCR) in reclaimed land | d) Nelumbo nucifera community (R) in freshwater wetland |
Discussion
1. Distribution of wetland plant communities
Freshwater wetland plants displayed ruderal and competitive adaptative strategies reflecting the ecological environment (Alahuhta et al., 2011; Bornette and Puijalon, 2011; Lee et al., 2020).
Sixty percent of the plant communities in sand dunes showed CR/CSR strategies (Costa et al., 1996; Elmas, 2017; Lee et al., 2020): Artemisia fukudo, Atriplex gmelinii, Carex kobomugi, Calystegia soldanella, Digitaria ciliaris, Linaria japonica, Messerschmidia sibirica, Oenothera bieenis, Salicornia europaea, Salsola komarovii, Suaeda glauca, and Suaeda maritima (Table 4). Twenty percent of the plant communities showed SC/CSR strategies, including Carex pumila, Imperata cylindrica var. koenigii, Limonium tetragonum, and Zoysia sinica. Increasing ruderality occurred in the latter part of the sand dune succession, and disturbance factors were partially increased by artificial pressure commonly caused by trampling and roads or paths in the back dunes (Ciccarelli, 2015; Lee et al., 2020). Distribution research on biotic factors and abiotic factors is very important for restoration and conservation plans considering environmental changes (Jung and Kim, 1998; Lee et al., 2020; Moreno-Casasola, 1988). To date, the vegetation establishment on coastal sand dunes has been related to salinity in the soil and seawater and soil moisture content (Figure 5).
The plants in salt marshes were allocated to CR, C, and SC strategies (Son et al., 2020). They displayed competition and disturbance adaptative strategies reflecting the ecological environment (Lee and Kim, 2018; Lee et al., 2016). In the study area, nine species showed CR strategies: Artemisia fukudo, Artemisia scoparis, Aster tripolium, Atriplex gmelini, Imperata cylindrica var. koenigii, Salicornia europaea, Suaeda japonica, Suaeda malacosperma, and Suaeda maritima (Figure 6). Some of them had rhizomes and lateral vegetative growth and in disturbed salt marshes (Son et al., 2020).
The plants in reclaimed lands were allocated to the factors related to R strategies such as R/CR and CR, as well as R/CSR, SR/CSR, and CR/CSR strategies (Figure 6) (Min and Kim, 2000; Yang et al., 2021; Zhu et al., 2014). They displayed reclamation adaptative strategies reflecting the ecological environment. In this area, seven species showed R/CR strategies: Aeschnomene indica, Chenopodium album var. centrorubrum, Lolium perenne, Lotus corniculatus var. japonicus, Phragmites communis, Portulaca oleracea, and Soncous brachyotus. Six species showed CR strategies: Bidens frondosa, Echinochloa crus-galli, Echinochloa oryzicola, Erigeron canadensis, Fimbristylis longispica, and Setaria viridis.
On other reclaimed lands, plant species were aligned according to the degree of desalinization (i.e., salinity levels). Species composition and vegetation profiles changed conspicuously over time and according to the topographical regions (Min and Kim 1999; Yang et al., 2021; Zhu et al., 2014). Regardless of the time after reclamation, halophytes and glycophytes coexisted on the sites where soil salt leaching was slow. Species association among plants was weak on reclaimed lands in the early stages. However, with time, the strength of species associations increased among halophytes and glycophytes, and the two groups were linked by salt-tolerant glycophytes. This resulted in a series of species associations on old reclaimed lands.
Previous studies have investigated plant succession and the interaction between soil and plants after reclamation on the west coast of South Korea (Figure 6) (Min and Kim, 2000; Yang et al., 2021). These studies included one natural tidal flat site (Namdong), and five sites that differed in the number of years since being reclaimed: Hyundai A (1 year (y)); Hyundai B (2 y); Jangdeog (8 y); Mado (12 y); and Baegseog (30 y) (Min and Kim, 2000). The number of plant species occurring at each site was 10, 9, 15, 30, 28, and 38, respectively. Based on the distribution ranges of the plants according to salt gradients and soil moisture content, and species associations and life forms, plant succession was divided into two sere groups, hydrarch and xerarch. The major species of the former were Phragmites communis and Typha angustata (Figure 5). Species associated with the latter were [Suaeda japonica] → [Salicornia herbacea, S. japonica, Atriplex subcordata, Suaeda asparagoides] → [Aster tripolium, Carex scabrifolia, Zoysia sinica, Limonium tetragonum] → [Artemisia scoparia, Calamagrostis epigeios, and Setaria viridis] → [Imperata cylindrica var. koenigii, Sonchus brachyotus, S. viridis] → [Aeschynomene indica, Lotus corniculatus var. japonicus, Trifolium repens, and other non-halophytes]. The progression of interactions between soil and plants through succession was influenced by salt leaching, increase in species richness and biomass, and increase in soil organic matter (Min and Kim, 2000; Yang et al., 2021).
2. CSR strategies of coastal wetland plants
In the present study, our objective was to identify the CSR strategies of coastal wetland plants. Plant factor measurements (Costa et al., 1996; Hodgson, et al. 1999; Pierce et al., 2013; Negreiros, 2014; Elmas, 2017) have been used for sand dunes, salt marshes, reclaimed land, and freshwater plant conservation in South Korea and other countries. Succession stages of four aquatic wetlands have C-SR-R strategy related to sand dunes, and types of wetlands which seawaters have great effects on the plant distribution, R/CR strategy to fresh water wetlands, and C-S-CS strategy in dominant species of aquatic wetlands (Figure 6). Two large species used C/SC and SC strategies, Nelumbo nucifera and Phragmites communis. Overall, CSR triangles may provide a useful scientific basis for protecting and restoring coastal wetlands and their valuable ecosystem services (Bertness and Shumway, 1993; Min and Kim, 1999, 2000; Kim, 2005; Kim et al., 2011; Zhu et al., 2014; Lee and Kim, 2018).
Ruderal plants play an important ecological role in disturbed environments. These plants are typically hardy, fast-growing species that are well-adapted to thrive in areas where natural vegetation has been removed or disrupted, such as construction sites, roadsides, abandoned fields (Grime, 1974; Barbour et al., 2021; OpenAI, 2024). 1) Ruderal plants often have deep or fibrous root systems that help to bind the soil, preventing erosion and reducing the loss of nutrients. 2) They are among the first species to colonize barren or disturbed lands, initiating the process of ecological succession. By creating shade, improving soil structure, and adding organic matter, they prepare the ground for more complex plant communities. 3) These plants contribute to the nutrient cycle by capturing nutrients from the environment and returning them to the soil as organic matter when they die and decompose. 4) Ruderal plants provide habitat and food for various organisms, including insects, birds, and small mammals. For example, their seeds can be a critical food source for birds in urban or degraded environments. Stress-tolerant plants play crucial roles in various ecosystems and agricultural practices. 1) Deep-rooted plants stabilize soil, particularly in arid or degraded lands, reducing erosion by wind or water. They can establish in harsh environments and pave the way for other species to grow, aiding in ecological succession. By thriving in challenging environments, they support specific niches and organisms dependent on them. 2) Stress-tolerant plants like grasses and shrubs serve as food for livestock in arid regions. Certain species are used for biofuel production, thriving in environments unsuitable for food crops. Some are sources of pharmaceuticals, fibers, or other valuable compounds. 3) Stress-tolerant plants are efficient at capturing carbon dioxide even harsh environments, contributing to climate change mitigation. Competitor plant role refers to the way a plant interacts with others in its ecosystem by competing for resources such as light, water, nutrients, and space. Plants with a strong competitor role typically dominate their environment and suppress others through various mechanisms. 1) They grow quickly to outcompete neighbors for light and nutrients. They often develop extensive root systems and dense canopies to overshadow others. They are efficient in resource acquisition and utilization. 2) Competitor plants play a critical role in shaping plant communities and driving ecological succession. Their dominance can influence biodiversity, soil health, and the availability of resources for other organisms.
Carbon-absorbing resources such as freshwater plants and halophytes play a critical role in mitigating climate change by reducing the amount of carbon dioxide (CO₂) in the atmosphere (Kim, 1994; Kim, 2012; Hoveka et al., 2016; Duarte et al., 2017; Griscom et al., 2017; OpenAI, 2024). These resources, often referred to as "carbon sinks," absorb more carbon than they release, helping to offset greenhouse gas emissions. Below are some key carbon-absorbing resources and their functions: 1) By reducing atmospheric CO₂, these resources help stabilize global temperatures. 2) Forests, wetlands, and oceans provide additional benefits, such as biodiversity, water purification, and flood protection. 3) Carbon sinks store carbon for long periods, reducing the immediate impact of emissions.
Conclusions
Plant species in freshwater wetlands were allocated to factors related to ruderality (R). The more abundant species in plant communities in sand dunes showed CR/CSR strategies. The plant species in salt marshes were allocated to CR, C, and SC strategies. The plant species in reclaimed lands were allocated to factors related to ruderal strategies such as R/CR and CR. The four habitat species were categorized by CSR classification. The strategies were different between seawater and freshwater habitats. The seawater-related plant species had similar C-SR-R strategies related to sand dunes, salt marshes, and reclaimed wetlands, whereas R/CR strategy was seen in plants in freshwater wetlands. Two large species such as Phragmites communis and Nelumbo nucifera showed different C-S-CS strategies related to later successions.
Acknowledgments
This research was supported by the Basic Science Research Program through the National Research Foundation of Korea (NRF) funded by the Ministry of Education of Korea (RS-2018-NR033226).
References
- Alahuhta, J., Heino, J., Luoto, M. 2011. Climate Change and the Future Distributions of Aquatic Macrophytes across Boreal Catchments. Journal of Biogeography, 38 (2): 383-393.
- Alvarez-Rogel, J., Jiménez-Cárceles F.J., Roca, M.J., Ortiz, R. 2007. Changes in soils and vegetation in a Mediterranean coastal salt marsh impacted by human activities. Estuarine Coastal and Shelf Science, 73(3-4): 510-526.
- Babour, H., Surmen, B., Kutbay H.G. 2021. Grime’s CSR strategies of aqutic macrophytes in different lagoon lakes Northern Turkey. Turkish Journal of Fisheries and Aquatic Science, 21: 211-224.
- Bertness M.D., Ellison, A.M., 1987. Determinants of pattern in a New England salt marsh plant community. Ecological Monographs, 57(2): 129-147.
- Bertness, M.D., Shumway, S.W., 1993. Competition and facilitation in marsh plants. American Naturalist, 142: 718–724.
- Bornette, G., Puijalon, S. 2011. Response of Aquatic Plants to Abiotic Factors: A Review. Aquatic Sciences, 73(1): 1–14.
- Castillo, S.A., Moreno-Casasola, P., 1996. Coastal sand dune vegetation: an extreme case of species invasion. Journal of Coastal Conservation, 2(1): 13-22.
- Cho, J.S., Lee, J.-S., Kim, J.-W., 2017. Distribution of Phragmites australis Communities with different habitat salinity. Journal of Coastal Research, 33(5): 1210-1216.
- Cho, K.H., Beon, M.-S, Jeong, J.J., 2018. Dynamics of soil salinity and vegetation in a reclaimed area in Saemangeum, Republic of Korea. Geoderma 321: 42-51.
- Ciccarelli, D., 2015. Mediterranean coastal dune vegetation: are disturbance and stress the key selective forces that drive the psammophilous succession. Estuarine, Coastal and Shelf Science, 165: 247–253.
- Costa, C.S.B., Cordazzo, C.V., Seeliger, U., 1996. Shore disturbance and dune plant distribution. Journal of Coastal Research, 12(1): 133-140.
- Duarte CM, Wu J, Xiao X, Bruhn A, Krause-Jensen D., 2017. Can seaweed farming play a role in climate change mitigation and adaptation? Frontiers in Marine Science, Volume 4.
- Elmas, E., 2017. Ecophysiological characteristics and CSR strategies of four dune species. Annales Botanici Fennici, 54(4-6): 353-360.
- Garbutt, A., Wolters, M., 2008. The natural regeneration of salt marsh on formerly reclaimed land. Applied Vegetation Science, 11(3): 335-344.
- Grime, J.P., 1974. Vegetation classification by reference to strategies. Nature, 250(5461): 26-31.
- Grime, J.P., 2002 Plant strategies, vegetation processes and ecosystem properties. Chichester, John Wiley and Sons, 222p.
- Griscom BW, Adams J, Ellis PW, Houghton RA., 2017. "Natural climate solutions." Proceedings of the National Academy of Sciences, 114(44): 11645–11650.
- Gu, J., Luo, M., Zhang, X., Christakos, G., Agusti, S., Duarte, C.M., Wu, J., 2018. Losses of salt marsh in China: Trends, threats and management. Estuarine, Coastal and Shelf Science 214: 98–109. doi: http://dx.doi.org/10.1016/j.ecss.2018.09.015.
- Hodgson, J.G., Wilson, P.J., Hunt, R., Grime, J.P., Thompson, K., 1999. Allocating C-S-R plant functional types: a soft approach to a hard problem. Oikos, 85(2): 282-294.
- Hong, S.-K., Koh, C.-H., Harris, R.R., Kim, J.E., Lee, J.-S., Ihm, B.-S. 2010. Land use in Korean tidal wetlands: management strategies. Environmental Management, 45: 1014-1026.
- Hoveka, L.N., Bezeng, B.S., Yessoufou, K., Boatwright, J.S., Van der Bank, M., 2016. Effects of climate change on the future distributions of the top five freshwater invasive plants in South Africa. South African Journal of Botany, 102: 33–38.
- Ihm, B.-S., Lee, J.-S. 1998. Soil factors affecting the plant communities of wetland on southwestern coast of Korea. Korean Journal of Ecology, 21(4): 321-328.
- Ihm, B.-S., Lee, J.-S., Kim, J.-W., Kim, J.-H., 2007. Coastal plant and soil relationships along the southwestern coast of South Korea. Journal of Plant Biology, 50(3): 331-335.
- Jung, Y.-K., Kim, J.-W., 1998. Coastal sand dune vegetation in Kyungpook province. Korean Journal of Ecology, 21(3): 257–262.
- Kim, C.S., 1983. Ecological studies on the halophyte communities at western and southern coasts in Korea(IV). Korean Journal of Ecology, 6(3): 167-176.
- Kim, D., Cairns, D.M., Bartholdy, J., 2011. Wind-driven sea-level variation influences dynamics of salt marsh vegetation. Annals of the Association of American Geographers, 101(2): 231-248.
- Kim, J.H. 2012. Gobal warming, Seoul National Univ. Pub.
- Kim, K.D., 2005. Invasive plants on disturbed Korean sand dunes. Estuarine, Coastal and Shelf Science, 62(1–2): 353–364.
- Lee, J.-S., Kim, J.-W., 2018. Dynamics of zonal halophyte communities in salt marshes in the world. Journal of Marine and Island Cultures, v7n1: 84-106.
- Lee, J.-S., Kim, J.-W., Lee, S.H., Myeong, H.-H., Lee, J.-Y., Cho, J.S., 2016. Zonation and soil factors of salt marsh halophyte communities. Journal of Ecology and Environment, 40:4. doi: 10.1186/s41610-016-0010-3.
- Lee, J.-S., Son, D.-H., Lee, S.-H., Myeong, H.-H., Cho, J.-S., Lee, J.-C., Lee, J.-Y., Park, C.-S., Kim, J.-W., 2020. Canonical correspondence analysis ordinations and competitor, stress tolerator, and ruderal strategies of coastal dune plants in South Korea. Journal of Coastal Research, 36(3): 528–535.
- Martinez, M.L., Maun, M.A., Psuty, N.P., 2008. The fragility and conservation of the world’s coastal dunes: Geomorphological, ecological and socioeconomic perspectives. In: Martinez, M.L., Psuty, N.P., (ed.) Coastal dunes, New York: Springer-Verlag, pp. 355-369.
- Min, B.M., Kim, J.-H., 1999. Plant distribution in relation to soil of reclaimed lands on the west coast of Korea. Journal of Plant Biology, 42: 287-293.
- Min, B.M., Kim, J.-H., 2000. Plant Succession and Interaction between Soil and Plants after Land Reclamation on the West Coast of Korea. Journal of Plant Biology, 43: 41-47.
- Moreno-Casasola, P., 1988. Patterns of plant species distribution on coastal dunes along the Gulf of Mexico. Journal of Biogeography, 15(5-6): 787–806.
- Myeong, H.H., 2010. On Conservation and Management Plan of Coastal Sand Dune Ecosystem using Health Assessment. Mokpo National University. Muan. Korea. Ph.D dissertation. 265p.
- Negreiros, D., Stradic, S.L., Fernandes, G.W., Renno, H.C., 2014. CSR analysis of plant functional types in highly diverse tropical grasslands of harsh environments. Plant Ecology, 215: 379–388.
- OpenAI, 2024. ChatGPT. CSR plant role or carbon absorbing resource function. https://chat.openai.com.
- Pierce, S., Brusa, G., Vagge, I., Cerabolini, B.E.L., 2013. Allocating CSR plant functional types: The use of leaf economics and size traits to classify woody and herbaceous vascular plants. Functional Ecology, 27(4): 1002–1010.
- Pierce, S., Luzzaro, A., Caccianiga, M., Ceriani, R.M., Cerabolini, B., 2007. Disturbance is the principal α-scale filter determining niche differentiation, coexistence and biodiversity in an alpine community. Journal of Ecology, 95(4): 698–706.
- Pomeroy, L.R., Wiegert, R.G., 1981. The ecology of a salt marsh. Ecological Studies Series, vol. 38. New York: Springer-Verlag .
- Rooney, N., Kalff, J., 2000. Inter-Annual Variation in Submerged Macrophyte Community Biomass and Distribution: The Influence of Temperature and Lake Morphometry. Aquatic Botany, 68(4): 321–335.
- Silander, J.A., Antonovics, J., 1982. Analysis of interspecific competition in a coastal plant community, a perturbation approach. Nature, 298: 557-560.
- Son, D.-H., Lee, J.-S., Kim, D.E., Lee, S.H., Hong, S.-K., Myeong, H.-H., Cho, J.-S., Lee, J.-C., Lee, J.-Y., Seon, H.J., Kim, J.-W., 2020. Importance of biotic and soil factors in determining the distribution strategies of coastal salt marsh plants. Journal of Marine and Island Cultures, v9n2. doi: 10.21463/jmic.2020.09.2.01.
- Suzuki, T., 2003. Economic and geographic backgrounds of land reclamation in Japanese ports. Marine Pollution Bulletin, 47(1-6): 226–229.
- Ter Braak, C.J.F., 1986. Canonical correspondence analysis: a new eigenvector technique for multivariate direct gradient analysis. Ecology, 67(5): 1167-1179.
- Ter Braak, C.J.F., Smilauer P., 2018. Canoco reference manual and user's guide: software for ordination (version 5.10). New York: Microcomputer Power, 536p.
- Yang, K.H., Park, S.B., Kim, S.Y., Cho, J.-S., Lee, J.-S, You C., Kim, J.-E., Ju, H.G., Lee, S.H., Hong, S.-K., Myeong, H.-H., Ko S.-Y., Choi, J.-W., Yang, H.-S., Kim, J.-W. 2020.. Distribution strategies of Vascular plants at the reclaimed lands at the Saemangum Sea Dike. Journal of Marine and Island Cultures, v10n2. doi: 10.21463/jmic.2021.10.2.04.
- Zhu, M.S., Sun, T., Shao, D.D., 2014. Impact of land reclamation on the evolution of shoreline change and nearshore vegetation distribution in Yangtze River Estuary. Wetlands, 36(1): 11-17.